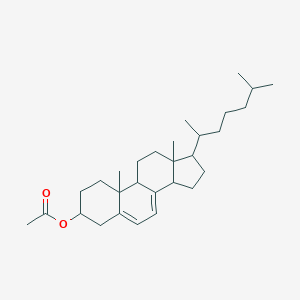
Acétate de 7-déhydrocholestérol
Vue d'ensemble
Description
7-Dehydrocholesterol acetate is a derivative of 7-dehydrocholesterol, a sterol found in animals. It is a precursor to vitamin D3, which is synthesized in the skin upon exposure to ultraviolet B radiation. This compound plays a crucial role in the biosynthesis of steroidal drugs and is widely used in various scientific and industrial applications .
Applications De Recherche Scientifique
7-Dehydrocholesterol acetate has a wide range of applications in scientific research:
Chemistry: It is used as an intermediate in the synthesis of various steroidal compounds.
Biology: The compound is crucial in studies related to cholesterol metabolism and vitamin D synthesis.
Medicine: It serves as a precursor for the production of vitamin D3, which is essential for bone health and immune function.
Industry: The compound is used in the production of feedstuffs and other industrial applications
Mécanisme D'action
Target of Action
7-Dehydrocholesterol acetate (7-DHC) is a sterol that functions as a precursor for several steroidal drugs . It primarily targets the ferroptosis pathway , a form of regulated cell death driven by iron-dependent lipid peroxidation . The key enzymes in the distal cholesterol synthesis pathway regulate the sensitivity to ferroptosis by controlling the levels of 7-DHC .
Mode of Action
7-DHC acts as an endogenous suppressor of ferroptosis . It exerts its action by transferring the peroxidation pathway of phospholipids, thereby protecting cells from phospholipid peroxidation on the plasma and mitochondrial membranes . This action reduces ferroptosis, a form of cell death that is associated with various diseases, including cancer, degenerative diseases, and organ ischemia-reperfusion injury (IRI) .
Biochemical Pathways
7-DHC is a metabolic intermediate in the cholesterol biosynthesis pathway . It is synthesized by the enzyme SC5D (sterol-C5-desaturase) and metabolized by the enzyme DHCR7 (7-dehydrocholesterol reductase) for cholesterol synthesis . As an unsaturated sterol, 7-DHC contains a 5,7-diene in its B-ring, which can act as a potent hydrogen atom donor for fatty acid peroxide free radicals .
Result of Action
The action of 7-DHC results in the protection of cells from ferroptosis . By controlling the levels of 7-DHC, cells can regulate their sensitivity to ferroptosis . This has significant therapeutic implications, as it suggests that controlling the levels of 7-DHC through drugs could be a promising strategy for treating cancer and IRI .
Action Environment
The action of 7-DHC can be influenced by environmental factors. For example, UV irradiation can directly convert 7-DHC to vitamin D3 . This conversion enables humans to manufacture vitamin D3, which is used to prevent and treat rickets and other bone diseases, improve immunity, and reduce the risk of cardiovascular disease . Therefore, the action, efficacy, and stability of 7-DHC can be influenced by factors such as exposure to sunlight.
Safety and Hazards
Handling of 7-Dehydrocholesterol acetate should be performed by personnel trained and familiar with handling of potent active pharmaceutical ingredients. It is recommended to avoid dust formation, breathing mist, gas or vapours, and contacting with skin and eye. Use of personal protective equipment and chemical impermeable gloves is advised .
Orientations Futures
Analyse Biochimique
Biochemical Properties
7-Dehydrocholesterol acetate interacts with various enzymes and proteins in the body. It is an intermediate in the synthesis of Zymostenol, which is a derivative of Zymosterol, an sterol intermediate in the biosynthesis of cholesterol . The enzyme 7-dehydrocholesterol reductase (DHCR7) plays a crucial role in its metabolism .
Cellular Effects
7-Dehydrocholesterol acetate has significant effects on various types of cells and cellular processes. It has been identified as a lipid-soluble antioxidant that protects cells from ferroptosis, a cell death pathway triggered by iron-catalyzed phospholipid peroxidation . This indicates that 7-Dehydrocholesterol acetate can influence cell function, including impacts on cell signaling pathways, gene expression, and cellular metabolism.
Molecular Mechanism
The molecular mechanism of 7-Dehydrocholesterol acetate involves its conversion into 7-Dehydrocholesterol by the Bamford-Stevens reaction . It exerts its effects at the molecular level, including binding interactions with biomolecules, enzyme inhibition or activation, and changes in gene expression .
Temporal Effects in Laboratory Settings
In laboratory settings, the effects of 7-Dehydrocholesterol acetate change over time. For instance, in a 96-hour shaker flask fermentation, the 7-Dehydrocholesterol titer was 649.5 mg/L by de novo synthesis . This indicates the product’s stability, degradation, and long-term effects on cellular function observed in in vitro studies.
Metabolic Pathways
7-Dehydrocholesterol acetate is involved in the cholesterol biosynthesis pathway . It interacts with enzymes such as lathosterol oxidase (lathosterol 5-desaturase), which is involved in the biosynthesis of cholesterol .
Transport and Distribution
It is known that cholesterol, a closely related compound, is predominantly localized to cell membranes, where it interacts with adjacent lipids to regulate rigidity, fluidity, and permeability of the bilayer .
Subcellular Localization
Related enzymes involved in sterol biosynthesis, such as DHCR24, have been found to be expressed in the endoplasmic reticulum and nuclear envelope .
Méthodes De Préparation
Synthetic Routes and Reaction Conditions: 7-Dehydrocholesterol acetate can be synthesized from 7-oxocholesterol acetate using the Bamford-Stevens reaction. This reaction involves the conversion of 7-oxocholesterol acetate to 7-dehydrocholesterol acetate with a yield of 79% based on the starting material .
Industrial Production Methods: In industrial settings, the production of 7-dehydrocholesterol acetate involves the use of advanced fermentation techniques. For instance, the biosynthesis pathway of 7-dehydrocholesterol has been reengineered in Saccharomyces cerevisiae, leading to significant improvements in yield and productivity .
Analyse Des Réactions Chimiques
Types of Reactions: 7-Dehydrocholesterol acetate undergoes various chemical reactions, including oxidation, reduction, and substitution.
Common Reagents and Conditions:
Oxidation: This reaction can be carried out using reagents such as chromium trioxide in the presence of acetic acid.
Reduction: Sodium borohydride is commonly used for the reduction of 7-dehydrocholesterol acetate.
Substitution: The compound can undergo substitution reactions with reagents like trichloroethylene.
Major Products: The major products formed from these reactions include various derivatives of 7-dehydrocholesterol, which are used in the synthesis of steroidal drugs and other bioactive compounds .
Comparaison Avec Des Composés Similaires
Ergosterol: Found in fungi, it is a precursor to vitamin D2.
Cholesterol: A major component of animal cell membranes and a precursor to steroid hormones.
Lathosterol: An intermediate in the biosynthesis of cholesterol.
Uniqueness: 7-Dehydrocholesterol acetate is unique due to its role as a direct precursor to vitamin D3, which is not the case for ergosterol or lathosterol. Its ability to be converted to vitamin D3 upon exposure to ultraviolet B radiation makes it particularly valuable in both biological and industrial contexts .
Propriétés
IUPAC Name |
[10,13-dimethyl-17-(6-methylheptan-2-yl)-2,3,4,9,11,12,14,15,16,17-decahydro-1H-cyclopenta[a]phenanthren-3-yl] acetate | |
---|---|---|
Details | Computed by Lexichem TK 2.7.0 (PubChem release 2021.05.07) | |
Source | PubChem | |
URL | https://pubchem.ncbi.nlm.nih.gov | |
Description | Data deposited in or computed by PubChem | |
InChI |
InChI=1S/C29H46O2/c1-19(2)8-7-9-20(3)25-12-13-26-24-11-10-22-18-23(31-21(4)30)14-16-28(22,5)27(24)15-17-29(25,26)6/h10-11,19-20,23,25-27H,7-9,12-18H2,1-6H3 | |
Details | Computed by InChI 1.0.6 (PubChem release 2021.05.07) | |
Source | PubChem | |
URL | https://pubchem.ncbi.nlm.nih.gov | |
Description | Data deposited in or computed by PubChem | |
InChI Key |
ACGNVBRAISEVDK-UHFFFAOYSA-N | |
Details | Computed by InChI 1.0.6 (PubChem release 2021.05.07) | |
Source | PubChem | |
URL | https://pubchem.ncbi.nlm.nih.gov | |
Description | Data deposited in or computed by PubChem | |
Canonical SMILES |
CC(C)CCCC(C)C1CCC2C1(CCC3C2=CC=C4C3(CCC(C4)OC(=O)C)C)C | |
Details | Computed by OEChem 2.3.0 (PubChem release 2021.05.07) | |
Source | PubChem | |
URL | https://pubchem.ncbi.nlm.nih.gov | |
Description | Data deposited in or computed by PubChem | |
Molecular Formula |
C29H46O2 | |
Details | Computed by PubChem 2.1 (PubChem release 2021.05.07) | |
Source | PubChem | |
URL | https://pubchem.ncbi.nlm.nih.gov | |
Description | Data deposited in or computed by PubChem | |
Molecular Weight |
426.7 g/mol | |
Details | Computed by PubChem 2.1 (PubChem release 2021.05.07) | |
Source | PubChem | |
URL | https://pubchem.ncbi.nlm.nih.gov | |
Description | Data deposited in or computed by PubChem | |
CAS No. |
1059-86-5 | |
Record name | Cholesta-5, acetate | |
Source | DTP/NCI | |
URL | https://dtp.cancer.gov/dtpstandard/servlet/dwindex?searchtype=NSC&outputformat=html&searchlist=226869 | |
Description | The NCI Development Therapeutics Program (DTP) provides services and resources to the academic and private-sector research communities worldwide to facilitate the discovery and development of new cancer therapeutic agents. | |
Explanation | Unless otherwise indicated, all text within NCI products is free of copyright and may be reused without our permission. Credit the National Cancer Institute as the source. | |
Retrosynthesis Analysis
AI-Powered Synthesis Planning: Our tool employs the Template_relevance Pistachio, Template_relevance Bkms_metabolic, Template_relevance Pistachio_ringbreaker, Template_relevance Reaxys, Template_relevance Reaxys_biocatalysis model, leveraging a vast database of chemical reactions to predict feasible synthetic routes.
One-Step Synthesis Focus: Specifically designed for one-step synthesis, it provides concise and direct routes for your target compounds, streamlining the synthesis process.
Accurate Predictions: Utilizing the extensive PISTACHIO, BKMS_METABOLIC, PISTACHIO_RINGBREAKER, REAXYS, REAXYS_BIOCATALYSIS database, our tool offers high-accuracy predictions, reflecting the latest in chemical research and data.
Strategy Settings
Precursor scoring | Relevance Heuristic |
---|---|
Min. plausibility | 0.01 |
Model | Template_relevance |
Template Set | Pistachio/Bkms_metabolic/Pistachio_ringbreaker/Reaxys/Reaxys_biocatalysis |
Top-N result to add to graph | 6 |
Feasible Synthetic Routes
Q1: What can you tell us about the reactivity of 7-Dehydrocholesterol acetate based on the provided research?
A1: One of the papers [] investigates the ozonolysis of 7-Dehydrocholesterol acetate peroxide. This reaction involves the cleavage of carbon-carbon double bonds by ozone. While the abstract doesn't detail the specific products or mechanism, it highlights the compound's reactivity towards ozone. This reactivity stems from the presence of double bonds in its structure, a characteristic feature of cholesterol derivatives.
Q2: What are the research papers about?
A2: The research papers focus on the chemical synthesis and reactions of 7-Dehydrocholesterol acetate:
- The first paper [] details a method for the "Synthesis of 7-dehydrocholesterol acetate." While the abstract doesn't provide specifics about the synthesis route, it implies the successful development of a procedure to obtain this compound.
- The second paper [] investigates the "Ozonolysis of 7-dehydrocholesterol acetate peroxide." This study likely explores the products and mechanism of the reaction between ozone and a peroxide derivative of 7-Dehydrocholesterol acetate.
Avertissement et informations sur les produits de recherche in vitro
Veuillez noter que tous les articles et informations sur les produits présentés sur BenchChem sont destinés uniquement à des fins informatives. Les produits disponibles à l'achat sur BenchChem sont spécifiquement conçus pour des études in vitro, qui sont réalisées en dehors des organismes vivants. Les études in vitro, dérivées du terme latin "in verre", impliquent des expériences réalisées dans des environnements de laboratoire contrôlés à l'aide de cellules ou de tissus. Il est important de noter que ces produits ne sont pas classés comme médicaments et n'ont pas reçu l'approbation de la FDA pour la prévention, le traitement ou la guérison de toute condition médicale, affection ou maladie. Nous devons souligner que toute forme d'introduction corporelle de ces produits chez les humains ou les animaux est strictement interdite par la loi. Il est essentiel de respecter ces directives pour assurer la conformité aux normes légales et éthiques en matière de recherche et d'expérimentation.