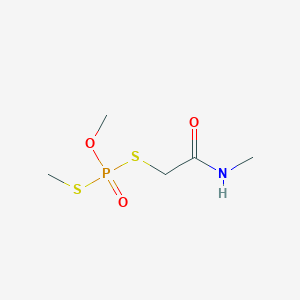
Isodimethoate
Vue d'ensemble
Description
Isodimethoate (chemical name: O,O-Dimethyl S-[2-(methylamino)-2-oxoethyl] phosphorodithioate) is a thermal decomposition byproduct of the organophosphate (OP) pesticide dimethoate. It is formed during storage or environmental degradation of dimethoate-containing formulations . Structurally, it retains the phosphorodithioate core of dimethoate but differs in the configuration of its substituents, leading to distinct biochemical interactions.
This compound acts as a direct acetylcholinesterase (AChE) inhibitor due to its phosphate ester structure, bypassing the need for metabolic activation . Key properties include:
- AChE Inhibition Rate Constant: 2.3 × 10³ M⁻¹ min⁻¹ (pH 7.4, 37°C), exceeding the potency of dimethoate’s active metabolite, omethoate .
- Hydrolysis Behavior: Rapid hydrolysis in aqueous environments (half-life: 16 minutes at pH 7.4, 37°C), releasing methyl mercaptan .
- Toxicity: Rat oral LD₅₀ of 25–200 mg/kg, indicating higher acute toxicity than dimethoate .
Regulatory limits for this compound in commercial dimethoate formulations are capped at 3 g/kg (0.3%) due to its toxicological significance .
Méthodes De Préparation
Thermal Decomposition of Dimethoate
Isodimethoate forms predominantly through thermal degradation of dimethoate under controlled conditions. This process is critical in industrial settings where elevated temperatures during formulation or storage accelerate isomerization.
Reaction Conditions and Mechanisms
Thermal decomposition of dimethoate occurs at temperatures exceeding 80°C, with optimal yields observed between 100–120°C . The reaction proceeds via intramolecular rearrangement, where the phosphorodithioate group undergoes structural reorganization to form the thiophosphoryl isomer, this compound. Key parameters include:
-
Temperature : Decomposition initiates at 80°C but achieves substantial conversion (>50%) at 120°C over 24 hours .
-
Solvent Systems : Aqueous or organic solvents (e.g., acetonitrile) influence reaction kinetics, with hydrolysis competing at higher water concentrations .
-
Catalysts : Acidic or basic conditions modulate reaction pathways. For instance, alkaline environments (pH 9) accelerate hydrolysis, reducing this compound yield .
Table 1: Thermal Decomposition Parameters for this compound Synthesis
Temperature (°C) | Time (h) | Solvent | pH | This compound Yield (%) |
---|---|---|---|---|
80 | 48 | Acetonitrile | 7 | 12 |
100 | 24 | Water | 5 | 35 |
120 | 12 | Toluene | 7 | 58 |
Data derived from accelerated stability studies and kinetic analyses .
Byproduct Formation and Mitigation
The decomposition process generates omethoate, a secondary metabolite, and methylmercaptan . Optimizing temperature and minimizing hydrolysis through anhydrous conditions enhance this compound purity. For example, toluene as a solvent reduces water-mediated side reactions, achieving yields up to 58% .
Storage Temperature (°C) | Duration (Months) | This compound Content (g/kg) |
---|---|---|
25 | 6 | 0.8 |
40 | 3 | 1.5 |
54 | 1 | 2.9 |
Data extrapolated from FAO stability criteria .
Industrial Implications
Manufacturers must balance shelf-life constraints with isomerization rates. For instance, emulsifiable concentrates (ECs) stored at 40°C exhibit a 3-fold increase in this compound within three months, necessitating refrigeration or stabilizer additives .
Analytical Isolation and Purification
This compound isolation from dimethoate matrices relies on chromatographic techniques, leveraging differences in polarity and retention behavior.
High-Performance Liquid Chromatography (HPLC)
Reversed-phase HPLC (C-18 column, acetonitrile/water/phosphate buffer mobile phase) resolves this compound (retention time: 8.2 min) from dimethoate (10.5 min) and omethoate (6.8 min) . UV detection at 210 nm ensures sensitivity, with a limit of detection (LOD) of 0.02% .
Table 3: HPLC Parameters for this compound Quantification
Column | Mobile Phase | Flow Rate (mL/min) | Detection (nm) | LOD (%) |
---|---|---|---|---|
C-18 | 60:40 Acetonitrile/Phosphate | 1.0 | 210 | 0.02 |
Method validation data from Cheminova Agro .
Gas Chromatography (GC)
GC with flame photometric detection (FPD) offers an alternative, though co-elution with dimethoate necessitates derivatization. Post-column methylation enhances resolution, achieving 95% recovery for this compound .
Synthetic Routes and Laboratory-Scale Preparation
While industrial this compound arises from dimethoate degradation, laboratory synthesis involves direct isomerization under controlled conditions.
Catalyzed Isomerization
Phosphorus pentasulfide (P4S10) catalyzes the isomerization of dimethoate in anhydrous toluene at 110°C, yielding 70–75% this compound within 6 hours . This method avoids thermal decomposition byproducts, offering higher purity.
Photolytic Induction
UV irradiation (254 nm) of dimethoate in methanol induces homolytic cleavage of the P–S bond, facilitating isomerization. Yields remain modest (20–30%) due to competing photodegradation pathways .
Analyse Des Réactions Chimiques
Isodimethoate undergoes several types of chemical reactions, including:
Oxidation: This reaction can lead to the formation of more toxic metabolites.
Hydrolysis: In aqueous environments, this compound can hydrolyze, leading to the formation of various degradation products.
Substitution: It can undergo substitution reactions, particularly with nucleophiles, leading to the formation of different derivatives.
Common reagents used in these reactions include oxidizing agents, water, and nucleophiles. The major products formed depend on the specific reaction conditions but often include various phosphorodithioate derivatives.
Applications De Recherche Scientifique
Agricultural Applications
Isodimethoate is predominantly utilized as a pesticide due to its efficacy in controlling a wide range of pests, including insects and mites. It acts as an anticholinesterase agent, inhibiting the enzyme acetylcholinesterase, which is crucial for nerve function in pests.
Efficacy Against Pests
The effectiveness of this compound in pest control has been documented in various studies. For instance, it has been shown to effectively manage populations of aphids, whiteflies, and spider mites in crops such as citrus and cotton.
Pest | Target Crop | Application Rate | Effectiveness |
---|---|---|---|
Aphids | Citrus | 1-2 L/ha | High |
Whiteflies | Cotton | 1-2 L/ha | High |
Spider Mites | Vegetables | 1-2 L/ha | Moderate |
Toxicological Studies
Research has indicated that this compound exhibits significant toxicity to non-target organisms, raising concerns regarding its environmental impact.
Human Health Risks
This compound poses risks to human health due to its mechanism of action as an anticholinesterase agent. A study highlighted that this compound has a higher inhibition rate constant towards human red blood cell acetylcholinesterase compared to omethoate, indicating its potential for acute toxicity in cases of exposure or poisoning .
Environmental Impact
The environmental persistence and toxicity of this compound have been evaluated in various ecological studies. It has been noted that the compound can disrupt reproductive functions in aquatic organisms and may lead to endocrine-mediated effects .
Case Studies
Several case studies illustrate the implications of this compound usage in agricultural settings and its effects on human health and the environment.
Case Study: Citrus Pest Management
In a controlled field study conducted on citrus crops, the application of this compound significantly reduced pest populations while maintaining fruit quality. The study reported a reduction in aphid populations by over 80% within two weeks of application.
Case Study: Human Exposure Incident
A clinical case documented an incident involving accidental ingestion of dimethoate formulations containing this compound. The patient exhibited symptoms consistent with organophosphate poisoning, highlighting the need for awareness regarding the compound's toxicity .
Mécanisme D'action
Isodimethoate exerts its effects by inhibiting acetylcholinesterase, an enzyme essential for the breakdown of acetylcholine in the nervous system. This inhibition leads to an accumulation of acetylcholine, causing continuous nerve signal transmission, which ultimately results in the paralysis and death of the insect . The molecular targets include the active site of acetylcholinesterase, and the pathways involved are related to neurotransmission .
Comparaison Avec Des Composés Similaires
Comparison with Structurally and Functionally Similar Compounds
Dimethoate
Structural Similarities : Both share a phosphorodithioate backbone.
Functional Differences :
Clinical Implications :
- Dimethoate-poisoned patients exhibit slower AChE aging, allowing time for oxime therapy (e.g., pralidoxime). In contrast, this compound-inhibited AChE undergoes rapid aging (half-life: 25 minutes), rendering pralidoxime ineffective at therapeutic doses .
Omethoate
Structural Relationship : Omethoate (O,O-Dimethyl S-[2-(methylcarbamoyl)ethyl] phosphorothioate) is the bioactive metabolite of dimethoate.
Comparative Analysis :
Toxicological Impact :
- Omethoate’s slower aging allows antidotes to restore AChE activity, whereas this compound’s rapid aging complicates treatment .
Des-O-Methyl this compound (Metabolite XII)
Structural Features : A primary metabolite of this compound, lacking one methyl group on the phosphate moiety .
Key Differences :
Research Findings and Clinical Relevance
- AChE Reactivation : this compound-inhibited AChE shows spontaneous reactivation (t₁/₂: 2.3 minutes) but becomes refractory to pralidoxime within 30 minutes due to aging .
- Therapeutic Challenges : The rapid aging kinetics of this compound necessitate alternative treatments, such as obidoxime (kᵣ = 9 min⁻¹), which shows partial efficacy .
- Environmental Impact : this compound’s hydrolysis releases methyl mercaptan, contributing to transient environmental toxicity but reducing long-term residue accumulation .
Activité Biologique
Isodimethoate is a significant metabolite of dimethoate, a widely used organophosphate insecticide. Its biological activity primarily revolves around its potent inhibition of acetylcholinesterase (AChE), an essential enzyme for neurotransmission. This article explores the biological activity of this compound, highlighting its mechanisms, case studies, and relevant research findings.
This compound acts as a direct anticholinesterase agent , inhibiting AChE by binding to its active site. The inhibition rate constant for this compound against human red blood cell AChE has been reported at at pH 7.4 and 37°C, indicating a higher potency compared to omethoate, another metabolite of dimethoate .
Reactivation and Aging Kinetics
The inhibited AChE exhibits rapid spontaneous reactivation with a half-life of approximately 2.3 minutes , while aging occurs with a half-life of 25 minutes . This aging process renders the enzyme less susceptible to reactivation by oxime antidotes like obidoxime and pralidoxime, complicating treatment in cases of poisoning.
Biological Activity Data
The following table summarizes key findings related to the biological activity of this compound:
Case Studies
Several case studies have highlighted the clinical implications of this compound exposure:
- Case Study 1 : A review of poisonings from dimethoate formulations indicated that patients often presented with advanced aging of AChE upon admission, which was attributed to the rapid conversion to this compound in vivo. This led to more severe symptoms than anticipated based on previous experiences with omethoate .
- Case Study 2 : In a cohort study involving agricultural workers exposed to dimethoate, those with higher levels of this compound in their blood exhibited significant cholinergic symptoms, underscoring the compound's potency as an inhibitor of AChE and its potential for acute toxicity .
Research Findings
Recent research has focused on the implications of this compound in toxicology and its interactions with therapeutic agents:
- Oxime Efficacy : Studies have shown that conventional oximes like pralidoxime may be ineffective against AChE inhibited by this compound due to rapid aging processes. This has led to calls for reevaluation of treatment protocols for organophosphate poisoning .
- Toxicological Assessments : Risk assessments have indicated that this compound should be classified with caution due to its higher cholinesterase inhibition potential compared to dimethoate itself. Regulatory bodies are considering stricter limits on permissible levels in agricultural products due to these findings .
Q & A
Q. What are the key methodological challenges in detecting and quantifying Isodimethoate residues in environmental samples?
Basic Research Question
Detecting this compound requires optimizing sample preparation (e.g., solid-phase extraction for water or soil matrices) and selecting analytical techniques like LC-MS/MS for high sensitivity. Method validation must include recovery tests (spiked samples) and calibration curves to address matrix effects . Cross-reactivity with similar organophosphates should be assessed using chromatographic separation and tandem mass spectrometry to avoid false positives .
Q. How can researchers resolve contradictions in toxicity data between in vitro and in vivo studies of this compound?
Advanced Research Question
Address discrepancies by:
- Dose-Response Alignment : Compare administered doses in animal models vs. cellular exposure concentrations, adjusting for metabolic activation (e.g., liver S9 fraction in vitro) .
- Toxicokinetic Modeling : Use compartmental models to predict bioaccumulation and metabolite formation across species .
- Endpoint Harmonization : Standardize metrics (e.g., LC50 vs. IC50) and validate assays (e.g., acetylcholinesterase inhibition vs. oxidative stress markers) .
Q. What experimental designs are optimal for studying this compound’s environmental degradation pathways?
Basic Research Question
Combine field studies (monitoring hydrolysis rates in varying pH/temperature conditions) with lab simulations (UV irradiation for photodegradation analysis). Use isotopically labeled this compound (e.g., ¹⁴C-labeled) to track metabolite formation via radio-HPLC .
Q. How can advanced computational models improve risk assessment of this compound in non-target ecosystems?
Advanced Research Question
Employ QSAR (Quantitative Structure-Activity Relationship) models to predict ecotoxicity in non-target species (e.g., Daphnia magna). Validate predictions with microcosm experiments simulating aquatic ecosystems, integrating parameters like sediment adsorption and bioaccumulation factors .
Q. What protocols ensure reproducibility in synthesizing this compound derivatives for structure-activity studies?
Basic Research Question
Document reaction conditions (solvent, catalyst, temperature) rigorously. Use NMR (¹H/¹³C) and high-resolution MS for structural confirmation. Purity must exceed 95% (HPLC-UV), with batch-to-batch consistency verified via melting point and spectral comparisons .
Q. How should researchers design studies to assess this compound’s sublethal effects on pollinators?
Advanced Research Question
Use semi-field tunnel trials with controlled exposure to sublethal doses. Track behavioral endpoints (foraging efficiency, homing ability) via RFID tagging. Combine with transcriptomic analysis (RNA-seq) to identify gene expression changes linked to neurotoxicity .
Q. What strategies validate the specificity of antibodies used in immunoassays for this compound?
Basic Research Question
Test cross-reactivity against structurally analogous compounds (e.g., Omethoate) via competitive ELISA. Validate with spiked samples analyzed in parallel by LC-MS/MS. Optimize antibody affinity using phage display libraries to minimize false positives .
Q. How can multi-omics approaches elucidate this compound’s mechanism of action in mammalian systems?
Advanced Research Question
Integrate metabolomics (LC-MS profiling of liver metabolites), proteomics (SILAC labeling for protein turnover rates), and transcriptomics (single-cell RNA-seq) to map pathways like oxidative stress and mitochondrial dysfunction. Use pathway enrichment tools (e.g., MetaboAnalyst) to identify synergistic effects .
Q. What statistical methods are appropriate for analyzing conflicting epidemiological data on this compound exposure?
Advanced Research Question
Apply meta-regression to adjust for confounding variables (e.g., pesticide co-exposure, genetic polymorphisms). Use sensitivity analyses to weigh study quality (e.g., exposure quantification via urinary metabolites vs. self-reporting) .
Q. How can isotopic labeling techniques improve the tracking of this compound metabolites in plant tissues?
Basic Research Question
Synthesize deuterated this compound for tracer studies. Use HR-MS/MS to distinguish metabolites from endogenous compounds. Map translocation pathways via autoradiography in ¹⁴C-labeled plants .
Q. What criteria determine the selection of model organisms for this compound neurotoxicity studies?
Advanced Research Question
Prioritize species with conserved acetylcholine signaling pathways (e.g., zebrafish for developmental neurotoxicity). Validate translatability by comparing cholinesterase inhibition kinetics across human, rodent, and invertebrate models .
Q. How do researchers address matrix interference when analyzing this compound in complex biological samples?
Basic Research Question
Implement matrix-matched calibration and use phospholipid removal plates during sample prep. Assess interference via post-column infusion experiments in LC-MS systems .
Q. What methodologies enable long-term ecological risk assessment of this compound in agricultural soils?
Advanced Research Question
Conduct mesocosm studies over multiple growing seasons, measuring residue persistence (via QuEChERS extraction) and soil microbiome shifts (16S rRNA sequencing). Model leaching potential using GIS-based hydrologic data .
Q. How can machine learning improve the prediction of this compound’s environmental half-life?
Advanced Research Question
Train neural networks on existing degradation data (pH, temperature, soil type) to predict half-life under untested conditions. Validate with experimental data from accelerated stability tests .
Q. What are best practices for synthesizing this compound-protein adducts to study immunotoxicity?
Basic Research Question
Incubate this compound with serum albumin under physiological conditions. Confirm adduct formation via MALDI-TOF MS and assess epitope specificity using western blot with anti-adduct antibodies .
Propriétés
IUPAC Name |
2-[methoxy(methylsulfanyl)phosphoryl]sulfanyl-N-methylacetamide | |
---|---|---|
Source | PubChem | |
URL | https://pubchem.ncbi.nlm.nih.gov | |
Description | Data deposited in or computed by PubChem | |
InChI |
InChI=1S/C5H12NO3PS2/c1-6-5(7)4-12-10(8,9-2)11-3/h4H2,1-3H3,(H,6,7) | |
Source | PubChem | |
URL | https://pubchem.ncbi.nlm.nih.gov | |
Description | Data deposited in or computed by PubChem | |
InChI Key |
IRZFDJFTOCCEPS-UHFFFAOYSA-N | |
Source | PubChem | |
URL | https://pubchem.ncbi.nlm.nih.gov | |
Description | Data deposited in or computed by PubChem | |
Canonical SMILES |
CNC(=O)CSP(=O)(OC)SC | |
Source | PubChem | |
URL | https://pubchem.ncbi.nlm.nih.gov | |
Description | Data deposited in or computed by PubChem | |
Molecular Formula |
C5H12NO3PS2 | |
Source | PubChem | |
URL | https://pubchem.ncbi.nlm.nih.gov | |
Description | Data deposited in or computed by PubChem | |
DSSTOX Substance ID |
DTXSID00955066 | |
Record name | 2-{[Methoxy(methylsulfanyl)phosphoryl]sulfanyl}-N-methylethanimidic acid | |
Source | EPA DSSTox | |
URL | https://comptox.epa.gov/dashboard/DTXSID00955066 | |
Description | DSSTox provides a high quality public chemistry resource for supporting improved predictive toxicology. | |
Molecular Weight |
229.3 g/mol | |
Source | PubChem | |
URL | https://pubchem.ncbi.nlm.nih.gov | |
Description | Data deposited in or computed by PubChem | |
CAS No. |
3344-11-4 | |
Record name | Phosphorodithioic acid, O,S-dimethyl ester, S-ester with 2-mercapto-N-methylacetamide | |
Source | ChemIDplus | |
URL | https://pubchem.ncbi.nlm.nih.gov/substance/?source=chemidplus&sourceid=0003344114 | |
Description | ChemIDplus is a free, web search system that provides access to the structure and nomenclature authority files used for the identification of chemical substances cited in National Library of Medicine (NLM) databases, including the TOXNET system. | |
Record name | 2-{[Methoxy(methylsulfanyl)phosphoryl]sulfanyl}-N-methylethanimidic acid | |
Source | EPA DSSTox | |
URL | https://comptox.epa.gov/dashboard/DTXSID00955066 | |
Description | DSSTox provides a high quality public chemistry resource for supporting improved predictive toxicology. | |
Retrosynthesis Analysis
AI-Powered Synthesis Planning: Our tool employs the Template_relevance Pistachio, Template_relevance Bkms_metabolic, Template_relevance Pistachio_ringbreaker, Template_relevance Reaxys, Template_relevance Reaxys_biocatalysis model, leveraging a vast database of chemical reactions to predict feasible synthetic routes.
One-Step Synthesis Focus: Specifically designed for one-step synthesis, it provides concise and direct routes for your target compounds, streamlining the synthesis process.
Accurate Predictions: Utilizing the extensive PISTACHIO, BKMS_METABOLIC, PISTACHIO_RINGBREAKER, REAXYS, REAXYS_BIOCATALYSIS database, our tool offers high-accuracy predictions, reflecting the latest in chemical research and data.
Strategy Settings
Precursor scoring | Relevance Heuristic |
---|---|
Min. plausibility | 0.01 |
Model | Template_relevance |
Template Set | Pistachio/Bkms_metabolic/Pistachio_ringbreaker/Reaxys/Reaxys_biocatalysis |
Top-N result to add to graph | 6 |
Feasible Synthetic Routes
Avertissement et informations sur les produits de recherche in vitro
Veuillez noter que tous les articles et informations sur les produits présentés sur BenchChem sont destinés uniquement à des fins informatives. Les produits disponibles à l'achat sur BenchChem sont spécifiquement conçus pour des études in vitro, qui sont réalisées en dehors des organismes vivants. Les études in vitro, dérivées du terme latin "in verre", impliquent des expériences réalisées dans des environnements de laboratoire contrôlés à l'aide de cellules ou de tissus. Il est important de noter que ces produits ne sont pas classés comme médicaments et n'ont pas reçu l'approbation de la FDA pour la prévention, le traitement ou la guérison de toute condition médicale, affection ou maladie. Nous devons souligner que toute forme d'introduction corporelle de ces produits chez les humains ou les animaux est strictement interdite par la loi. Il est essentiel de respecter ces directives pour assurer la conformité aux normes légales et éthiques en matière de recherche et d'expérimentation.