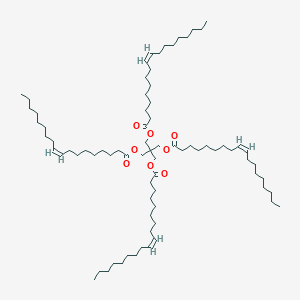
Pentaerythritol tetraoleate
Vue d'ensemble
Description
Pentaerythritol tetraoleate (PETO) is a tetraester derived from pentaerythritol and oleic acid. Its symmetrical structure, featuring four oleate chains attached to a central carbon atom (C(CH₂OOCR)₄), confers unique physicochemical properties, including high lubricity, thermal stability (>300°C flash point), biodegradability, and a viscosity index ≥180 . These attributes make PETO a critical component in industrial lubricants (e.g., ISO 68-grade hydraulic fluids, VG-68 engine oils), aviation turbine oils, and cosmetic emollients . Synthesized via esterification using acidic catalysts like p-toluenesulfonic acid, PETO achieves >95% purity after purification steps such as reduced-pressure distillation . Key physical properties include a viscosity of 60–70 mm²/s at 40°C, a pour point ≤-25°C, and an acid value ≤1 mg KOH/g .
Méthodes De Préparation
Transesterification of High Oleic Methyl Esters
Transesterification between high oleic palm oil methyl ester (POME) and pentaerythritol (PE) is a widely studied method for PETO synthesis. Sodium methoxide (NaOCH₃) serves as an effective catalyst, with vacuum conditions critical for removing methanol byproducts and shifting equilibrium toward ester formation .
Optimal Reaction Conditions
Key parameters for maximizing PETO yield include:
-
Temperature : 160°C
-
Catalyst concentration : 1.25% (w/w relative to PE)
-
Molar ratio (POME:PE) : 4.5:1
-
Vacuum pressure : 10 mbar
-
Stirring speed : 900 rpm
Under these conditions, a yield of 36% (w/w) is achieved within 2 hours . The reaction proceeds via nucleophilic acyl substitution, where methoxide ions deprotonate PE hydroxyl groups, enabling nucleophilic attack on POME carbonyl carbons.
Table 1: Transesterification Performance Under Varied Conditions
Parameter | Range Tested | Optimal Value | Yield (%) |
---|---|---|---|
Temperature (°C) | 140–180 | 160 | 36 |
Catalyst (%, w/w) | 0.5–2.0 | 1.25 | 36 |
Molar Ratio (POME:PE) | 3.5:1–5.0:1 | 4.5:1 | 36 |
Vacuum (mbar) | 5–15 | 10 | 36 |
Direct Esterification of Oleic Acid and Pentaerythritol
Direct esterification avoids toxic solvents like toluene by using nitrogen pressurization to suppress oleic acid oxidation. The reaction follows:
Industrial-Scale Protocol
-
Reactants : Oleic acid and PE at a 4:1 molar ratio.
-
Catalyst : Organotin compounds (e.g., Fascat 2003) at 0.5–1.0% (w/w).
-
Conditions :
-
Temperature: 180–220°C
-
Nitrogen pressure: 0.5–3 kg/cm²
-
Duration: 7–18 hours
-
Reaction completion is determined by acid value (<1 mg KOH/g). Post-reaction, crude PETO is washed with potassium phosphate solution to neutralize residual acid and dehydrated under reduced pressure .
Table 2: Direct Esterification Efficiency
Acid Value (mg KOH/g) | Reaction Time (h) | Yield (%) |
---|---|---|
5.2 | 7 | 62 |
2.1 | 12 | 78 |
0.8 | 18 | 89 |
Catalytic Systems and Kinetic Enhancements
Tin-Based Catalysts
Stannous oxide (SnO) and butyl stannic oxide significantly accelerate esterification:
-
SnO (0.46% w/w) : Achieves 99.8% PE conversion at 150–198°C within 4 hours .
-
Activation energy reduction : Tin’s Lewis acidity lowers the energy barrier for acyl-oxygen cleavage, increasing reaction rates 3-fold compared to sulfuric acid .
Acidic vs. Basic Catalysts
-
Sulfuric acid : Generates sulfonated byproducts, requiring intensive post-washing .
-
Sodium methoxide : Higher selectivity but necessitates anhydrous conditions .
Table 3: Catalyst Performance Comparison
Catalyst | Temperature (°C) | Time (h) | Conversion (%) |
---|---|---|---|
SnO | 150–198 | 4 | 99.8 |
NaOCH₃ | 160 | 2 | 95.4 |
H₂SO₄ | 180 | 7 | 91.2 |
Purification and Quality Control
Solvent-Free Dehydration
Industrial processes use reduced-pressure distillation (10–50 mbar) to remove water, achieving PETO purity >95% . Residual acidity is neutralized with 10% potassium carbonate, yielding a final acid value ≤0.5 mg KOH/g .
Chromatographic Analysis
Gas chromatography (GC) quantifies tri- and tetraester content, with optimal batches containing 97.4% tetraesters . Raman spectroscopy confirms ester linkage formation via C=O stretching at 1740 cm⁻¹ .
Industrial Production Workflows
Batch Reactor Configuration
-
Reactor type : Glass-lined steel with anchor agitators (900 rpm).
-
Heating : Thermal oil jackets maintain ±2°C temperature uniformity.
-
Byproduct management : Condensers recover oleic acid vapors for reuse.
Continuous Process Development
Pilot-scale studies demonstrate that tubular reactors with static mixers reduce reaction time to 45 minutes at 200°C and 20 bar .
Environmental and Economic Considerations
Solvent-Free Advantages
Direct esterification eliminates 300–500 kg/hr of toluene emissions per facility, reducing VOC compliance costs by 18% .
Feedstock Optimization
Using recycled oleic acid from food industry waste lowers raw material costs by 22% without compromising PETO viscosity (60–70 mm²/s at 40°C) .
Analyse Des Réactions Chimiques
Types of Reactions: Pentaerythritol tetraoleate primarily undergoes esterification and transesterification reactions. It can also participate in oxidation and hydrolysis reactions under specific conditions.
Common Reagents and Conditions:
Esterification: Oleic acid and pentaerythritol in the presence of an acidic catalyst.
Transesterification: Reaction with other alcohols or esters in the presence of a base catalyst.
Oxidation: Exposure to oxidizing agents like hydrogen peroxide under controlled conditions.
Hydrolysis: Reaction with water or aqueous solutions of acids or bases.
Major Products Formed:
Esterification: this compound.
Transesterification: Various esters depending on the alcohol or ester used.
Oxidation: Oxidized derivatives of this compound.
Hydrolysis: Pentaerythritol and oleic acid.
Applications De Recherche Scientifique
Lubrication and Hydraulic Fluids
Properties and Performance:
PETO exhibits excellent lubricating properties, high flash points (272°C), and low volatility, making it suitable for use in fire-resistant hydraulic fluids and lubricants for industrial applications. Its biodegradability rate exceeds 90%, which is advantageous for environmentally friendly formulations .
Case Study: Industrial Gear Oil
A study demonstrated that PETO could be utilized as an industrial gear oil. The ester showed superior fire resistance compared to conventional oils, making it a preferred choice in high-temperature environments .
Table 1: Performance Characteristics of this compound
Property | Value |
---|---|
Flash Point | 272°C |
Viscosity Index | High |
Biodegradation Rate | >90% |
Pour Point | -32°C |
Food Technology
Biotechnological Applications:
PETO has been investigated as a potential ingredient for low-calorie food alternatives. Research indicates that it can serve as a healthier substitute for butter and sweeteners due to its low toxicity and favorable hydrolysis properties when catalyzed by lipases .
Case Study: Hydrolysis for Food Applications
In a kinetic analysis of lipase-catalyzed hydrolysis, PETO was shown to release fatty acids effectively, making it suitable for developing low-calorie food products. The study provided insights into the pKa values of released fatty acids, which are crucial for understanding their behavior in food matrices .
Synthesis and Chemical Reactions
Transesterification Processes:
PETO can be synthesized from high oleic palm oil methyl ester (POME) through transesterification. This process has been optimized to yield PETO effectively under specific conditions (e.g., temperature of 160°C, catalyst concentration of 1.25%, etc.) .
Table 2: Optimal Conditions for Synthesis of this compound
Parameter | Optimal Value |
---|---|
Reaction Temperature | 160°C |
Catalyst Concentration | 1.25% (w/w) |
Molar Ratio (POME:PE) | 4.5:1 |
Vacuum Pressure | 10 mbar |
Stirring Speed | 900 rpm |
Environmental Impact and Safety
Toxicological Assessments:
Toxicity studies have shown that PETO is non-genotoxic and does not adversely affect reproductive parameters in animal models. This safety profile supports its use in consumer products, including cosmetics and food .
Mécanisme D'action
The primary mechanism of action of pentaerythritol tetraoleate in its applications is its ability to form a lubricating film on surfaces, reducing friction and wear. This is due to its high viscosity and ability to adhere to metal surfaces. In biological systems, its biodegradability allows it to be broken down into non-toxic components, making it suitable for eco-friendly applications .
Comparaison Avec Des Composés Similaires
Trimethylolpropane (TMP) Dimer Tetraoleate
- Structure and Composition : TMP dimer, like PETO, contains four hydroxyl groups and forms tetraoleate esters. Its tri- and tetraester content (97.4 wt%) closely matches PETO’s ester profile .
- Synthesis : Both compounds use homogeneous metallic catalysts (e.g., sulfonic acids) under similar conditions (50°C, 3–6 hours). However, TMP dimer’s triester content peaks earlier (20 wt% at 3 hours) before declining, suggesting kinetic differences in esterification .
- Applications : Used in fire-resistant hydraulic fluids and metalworking oils, TMP dimer tetraoleate exhibits comparable lubricity but may differ in oxidative stability due to structural variations .
Neopentyl Glycol Dioleate (NPG) and Trimethylolpropane Trioleate (TMP Trioleate)
- Ester Functionality : NPG (two ester groups) and TMP trioleate (three esters) have lower branching than PETO, resulting in reduced thermal stability and viscosity. For example, TMP trioleate has a viscosity ~30–40 mm²/s at 40°C, significantly lower than PETO’s 60–70 mm²/s .
- Performance : These esters are suitable for low-pressure lubricants but lack PETO’s high-temperature resilience. NPG’s simpler structure also limits its use in aviation-grade oils .
Pentaerythritol Tetrastearate and Erythritol Tetraoleate
- Fatty Acid Chain Impact: Replacing oleic acid (monounsaturated C18:1) with stearic acid (saturated C18:0) in pentaerythritol tetrastearate increases melting point but reduces oxidative stability. Hydrolysis studies show pentaerythritol tetrastearate releases stearic acid with pKa values (4.54–7.76), contrasting PETO’s oleic acid (pKa 5.12) .
- Biotechnological Use: Erythritol tetraoleate, a PETO analog with a shorter carbon backbone, is explored as a low-calorie food additive due to slower enzymatic hydrolysis rates (e.g., Candida antarctica lipase B activity) .
Pentaerythritol Tetra(2-ethylhexanoate)
- Branching Effects: The shorter, branched 2-ethylhexanoate chains lower viscosity and improve low-temperature performance (e.g., pour point <-40°C) but sacrifice lubricity at high loads. This makes it ideal for cold-climate applications but less suited for industrial gear oils .
Cosmetic Analogs: Pentaerythritol Tetraisostearate
- Functional Differences : Tetraisostearate’s iso-stearic acid chains enhance gel-forming capacity in cosmetics, offering a thicker texture compared to PETO’s liquid emollient properties .
Data Tables
Table 1: Key Properties of PETO and Analogous Esters
Table 2: Hydrolysis Behavior of Tetraesters
Research Findings and Industrial Implications
- Synthetic Efficiency : PETO’s synthesis achieves >95% purity with minimal byproducts, outperforming TMP dimer’s triester variability .
- Thermal Stability : PETO’s flash point (>300°C) exceeds most analogs, making it indispensable in high-temperature machinery .
- Environmental Impact : PETO’s biodegradability aligns with eco-friendly lubricant trends, though TMP dimer esters offer similar sustainability .
Activité Biologique
Pentaerythritol tetraoleate (PETO) is a synthetic ester derived from pentaerythritol and oleic acid, notable for its unique properties and applications in various industrial sectors. This article delves into the biological activity of PETO, examining its synthesis, properties, and potential applications, supported by research findings and data tables.
Synthesis of this compound
PETO is synthesized through the esterification of pentaerythritol with oleic acid. The reaction typically requires specific catalysts and controlled conditions to achieve high yields. For instance, studies have shown that using sodium methoxide as a catalyst under vacuum conditions can optimize the transesterification process. The ideal reaction parameters include:
- Temperature: 160 °C
- Catalyst Concentration: 1.25% (w/w)
- Molar Ratio (POME to PE): 4.5:1
- Vacuum Pressure: 10 mbar
- Stirring Speed: 900 rpm
Under these conditions, a yield of approximately 36% (w/w) of this compound can be achieved within two hours .
PETO exhibits several advantageous properties:
- High Lubricating Properties: PETO serves as an effective base oil for synthetic lubricants due to its excellent lubricity.
- Viscosity Index: It has a high viscosity index, making it suitable for applications requiring consistent performance across varying temperatures.
- Biodegradability: PETO is biodegradable, which enhances its appeal in environmentally conscious applications.
- Flame Retardancy: Its flame-retardant characteristics make it suitable for use in fire-resistant hydraulic fluids .
Toxicological Studies
Research indicates that PETO has a favorable safety profile. In toxicity studies involving Sprague-Dawley rats, no significant adverse effects were observed at high doses (up to 5000 mg/kg). The compound exhibited minimal skin irritation and did not affect reproductive organs or cause teratogenic effects at doses up to 2000 mg/kg .
Case Studies
- Biolubricant Applications:
- Hydraulic Fluids:
Research Findings
The following table summarizes key findings from various studies on the synthesis and properties of this compound:
Q & A
Basic Research Questions
Q. What are the optimal reaction conditions for synthesizing PETO via transesterification?
PETO is synthesized via transesterification of high oleic palm oil methyl ester (POME) with pentaerythritol (PE) using sodium methoxide as a catalyst. Key parameters include:
- Temperature : 160 °C
- Catalyst concentration : 1.25% (w/w)
- Molar ratio (POME:PE) : 4.5:1
- Vacuum pressure : 10 mbar
- Stirring speed : 900 rpm Under these conditions, a yield of 36% (w/w) is achieved within 2 hours. Vacuum application removes methanol byproducts, shifting equilibrium toward ester formation .
Q. What analytical methods are used to characterize PETO’s purity and structural properties?
- Gas Chromatography (GC) : Quantifies triester and tetraester content during synthesis (e.g., 97.4% total tri- and tetraesters after purification) .
- Raman Spectroscopy and TEM : Detect friction-induced graphene-like layered structures on metal surfaces, correlating with enhanced lubrication .
- Tribological Testing : Measures wear volume and torque (e.g., PETO reduced wear on 316 stainless steel at 2 N load and 500 rpm) .
Q. How is PETO’s tribological performance evaluated in metal processing applications?
PETO’s performance is tested using tapping torque tests on materials like Al 6061. Metrics include:
- Torque vs. depth of cut : PETO outperforms other polyol esters (e.g., TMP-TO, NPG-DO) due to superior lubrication .
- Wear volume quantification : Compared to dry friction, PETO reduces wear by forming protective surface layers .
Advanced Research Questions
Q. What factors limit PETO synthesis yield, and how can they be addressed?
Despite optimized conditions, yields remain low (~36–37.56%) due to:
- Reverse reactions : Methanol byproduct accumulation inhibits forward progression. Enhanced vacuum systems or continuous methanol removal could improve yield .
- Catalyst efficiency : Sodium methoxide may degrade at high temperatures. Alternative catalysts (e.g., lipases) or immobilized systems could enhance stability .
Q. What mechanisms underlie PETO’s friction-induced formation of graphene-like layers?
Under shear stress, PETO interacts with polyalphaolefin (PAO4) to generate tribofilms via:
- Carbon reorganization : Raman spectra confirm sp²-hybridized carbon structures resembling graphene.
- TEM imaging : Reveals lamellar layers (1–5 nm thick) on 316 stainless steel, reducing friction coefficients by 40–60% . Methodological approach: Use ball-on-disk tribometry coupled with post-test surface characterization (Raman, TEM).
Q. How does PETO synergize with PAO4 to enhance lubrication?
PETO and PAO4 exhibit synergistic lubrication via:
- Polar-nonpolar interactions : PETO’s ester groups adsorb onto metal surfaces, while PAO4’s hydrocarbon chains reduce shear stress.
- Load-bearing capacity : At 10 N load, the PETO-PAO4 blend reduces wear volume by 50% compared to individual components . Experimental design: Compare friction/wear performance of blends vs. individual lubricants under varying loads (2–10 N).
Q. How does ester composition (tri- vs. tetraesters) influence PETO’s properties?
- GC analysis : Post-synthesis purification reveals ~5% triester and ~92% tetraester content. Higher tetraester content improves thermal stability (flash point >300 °C) and viscosity index (≥180) .
- Tribological impact : Tetraesters form more ordered surface films, enhancing load capacity. Triesters may reduce oxidative stability due to free hydroxyl groups .
Propriétés
IUPAC Name |
[3-[(Z)-octadec-9-enoyl]oxy-2,2-bis[[(Z)-octadec-9-enoyl]oxymethyl]propyl] (Z)-octadec-9-enoate | |
---|---|---|
Source | PubChem | |
URL | https://pubchem.ncbi.nlm.nih.gov | |
Description | Data deposited in or computed by PubChem | |
InChI |
InChI=1S/C77H140O8/c1-5-9-13-17-21-25-29-33-37-41-45-49-53-57-61-65-73(78)82-69-77(70-83-74(79)66-62-58-54-50-46-42-38-34-30-26-22-18-14-10-6-2,71-84-75(80)67-63-59-55-51-47-43-39-35-31-27-23-19-15-11-7-3)72-85-76(81)68-64-60-56-52-48-44-40-36-32-28-24-20-16-12-8-4/h33-40H,5-32,41-72H2,1-4H3/b37-33-,38-34-,39-35-,40-36- | |
Source | PubChem | |
URL | https://pubchem.ncbi.nlm.nih.gov | |
Description | Data deposited in or computed by PubChem | |
InChI Key |
QTIMEBJTEBWHOB-PMDAXIHYSA-N | |
Source | PubChem | |
URL | https://pubchem.ncbi.nlm.nih.gov | |
Description | Data deposited in or computed by PubChem | |
Canonical SMILES |
CCCCCCCCC=CCCCCCCCC(=O)OCC(COC(=O)CCCCCCCC=CCCCCCCCC)(COC(=O)CCCCCCCC=CCCCCCCCC)COC(=O)CCCCCCCC=CCCCCCCCC | |
Source | PubChem | |
URL | https://pubchem.ncbi.nlm.nih.gov | |
Description | Data deposited in or computed by PubChem | |
Isomeric SMILES |
CCCCCCCC/C=C\CCCCCCCC(=O)OCC(COC(=O)CCCCCCC/C=C\CCCCCCCC)(COC(=O)CCCCCCC/C=C\CCCCCCCC)COC(=O)CCCCCCC/C=C\CCCCCCCC | |
Source | PubChem | |
URL | https://pubchem.ncbi.nlm.nih.gov | |
Description | Data deposited in or computed by PubChem | |
Molecular Formula |
C77H140O8 | |
Source | PubChem | |
URL | https://pubchem.ncbi.nlm.nih.gov | |
Description | Data deposited in or computed by PubChem | |
DSSTOX Substance ID |
DTXSID80893371 | |
Record name | Pentaerythrityl tetraoleate | |
Source | EPA DSSTox | |
URL | https://comptox.epa.gov/dashboard/DTXSID80893371 | |
Description | DSSTox provides a high quality public chemistry resource for supporting improved predictive toxicology. | |
Molecular Weight |
1193.9 g/mol | |
Source | PubChem | |
URL | https://pubchem.ncbi.nlm.nih.gov | |
Description | Data deposited in or computed by PubChem | |
Physical Description |
Liquid | |
Record name | 9-Octadecenoic acid (9Z)-, 1,1'-[2,2-bis[[[(9Z)-1-oxo-9-octadecenyl]oxy]methyl]-1,3-propanediyl] ester | |
Source | EPA Chemicals under the TSCA | |
URL | https://www.epa.gov/chemicals-under-tsca | |
Description | EPA Chemicals under the Toxic Substances Control Act (TSCA) collection contains information on chemicals and their regulations under TSCA, including non-confidential content from the TSCA Chemical Substance Inventory and Chemical Data Reporting. | |
CAS No. |
19321-40-5 | |
Record name | Pentaerythrityl tetraoleate | |
Source | CAS Common Chemistry | |
URL | https://commonchemistry.cas.org/detail?cas_rn=19321-40-5 | |
Description | CAS Common Chemistry is an open community resource for accessing chemical information. Nearly 500,000 chemical substances from CAS REGISTRY cover areas of community interest, including common and frequently regulated chemicals, and those relevant to high school and undergraduate chemistry classes. This chemical information, curated by our expert scientists, is provided in alignment with our mission as a division of the American Chemical Society. | |
Explanation | The data from CAS Common Chemistry is provided under a CC-BY-NC 4.0 license, unless otherwise stated. | |
Record name | Pentaerythritol tetraoleate | |
Source | ChemIDplus | |
URL | https://pubchem.ncbi.nlm.nih.gov/substance/?source=chemidplus&sourceid=0019321405 | |
Description | ChemIDplus is a free, web search system that provides access to the structure and nomenclature authority files used for the identification of chemical substances cited in National Library of Medicine (NLM) databases, including the TOXNET system. | |
Record name | 9-Octadecenoic acid (9Z)-, 1,1'-[2,2-bis[[[(9Z)-1-oxo-9-octadecenyl]oxy]methyl]-1,3-propanediyl] ester | |
Source | EPA Chemicals under the TSCA | |
URL | https://www.epa.gov/chemicals-under-tsca | |
Description | EPA Chemicals under the Toxic Substances Control Act (TSCA) collection contains information on chemicals and their regulations under TSCA, including non-confidential content from the TSCA Chemical Substance Inventory and Chemical Data Reporting. | |
Record name | Pentaerythrityl tetraoleate | |
Source | EPA DSSTox | |
URL | https://comptox.epa.gov/dashboard/DTXSID80893371 | |
Description | DSSTox provides a high quality public chemistry resource for supporting improved predictive toxicology. | |
Record name | Pentaerythritol tetraoleate | |
Source | European Chemicals Agency (ECHA) | |
URL | https://echa.europa.eu/substance-information/-/substanceinfo/100.039.039 | |
Description | The European Chemicals Agency (ECHA) is an agency of the European Union which is the driving force among regulatory authorities in implementing the EU's groundbreaking chemicals legislation for the benefit of human health and the environment as well as for innovation and competitiveness. | |
Explanation | Use of the information, documents and data from the ECHA website is subject to the terms and conditions of this Legal Notice, and subject to other binding limitations provided for under applicable law, the information, documents and data made available on the ECHA website may be reproduced, distributed and/or used, totally or in part, for non-commercial purposes provided that ECHA is acknowledged as the source: "Source: European Chemicals Agency, http://echa.europa.eu/". Such acknowledgement must be included in each copy of the material. ECHA permits and encourages organisations and individuals to create links to the ECHA website under the following cumulative conditions: Links can only be made to webpages that provide a link to the Legal Notice page. | |
Record name | PENTAERYTHRITYL TETRAOLEATE | |
Source | FDA Global Substance Registration System (GSRS) | |
URL | https://gsrs.ncats.nih.gov/ginas/app/beta/substances/TR8SGI88PN | |
Description | The FDA Global Substance Registration System (GSRS) enables the efficient and accurate exchange of information on what substances are in regulated products. Instead of relying on names, which vary across regulatory domains, countries, and regions, the GSRS knowledge base makes it possible for substances to be defined by standardized, scientific descriptions. | |
Explanation | Unless otherwise noted, the contents of the FDA website (www.fda.gov), both text and graphics, are not copyrighted. They are in the public domain and may be republished, reprinted and otherwise used freely by anyone without the need to obtain permission from FDA. Credit to the U.S. Food and Drug Administration as the source is appreciated but not required. | |
Retrosynthesis Analysis
AI-Powered Synthesis Planning: Our tool employs the Template_relevance Pistachio, Template_relevance Bkms_metabolic, Template_relevance Pistachio_ringbreaker, Template_relevance Reaxys, Template_relevance Reaxys_biocatalysis model, leveraging a vast database of chemical reactions to predict feasible synthetic routes.
One-Step Synthesis Focus: Specifically designed for one-step synthesis, it provides concise and direct routes for your target compounds, streamlining the synthesis process.
Accurate Predictions: Utilizing the extensive PISTACHIO, BKMS_METABOLIC, PISTACHIO_RINGBREAKER, REAXYS, REAXYS_BIOCATALYSIS database, our tool offers high-accuracy predictions, reflecting the latest in chemical research and data.
Strategy Settings
Precursor scoring | Relevance Heuristic |
---|---|
Min. plausibility | 0.01 |
Model | Template_relevance |
Template Set | Pistachio/Bkms_metabolic/Pistachio_ringbreaker/Reaxys/Reaxys_biocatalysis |
Top-N result to add to graph | 6 |
Feasible Synthetic Routes
Avertissement et informations sur les produits de recherche in vitro
Veuillez noter que tous les articles et informations sur les produits présentés sur BenchChem sont destinés uniquement à des fins informatives. Les produits disponibles à l'achat sur BenchChem sont spécifiquement conçus pour des études in vitro, qui sont réalisées en dehors des organismes vivants. Les études in vitro, dérivées du terme latin "in verre", impliquent des expériences réalisées dans des environnements de laboratoire contrôlés à l'aide de cellules ou de tissus. Il est important de noter que ces produits ne sont pas classés comme médicaments et n'ont pas reçu l'approbation de la FDA pour la prévention, le traitement ou la guérison de toute condition médicale, affection ou maladie. Nous devons souligner que toute forme d'introduction corporelle de ces produits chez les humains ou les animaux est strictement interdite par la loi. Il est essentiel de respecter ces directives pour assurer la conformité aux normes légales et éthiques en matière de recherche et d'expérimentation.