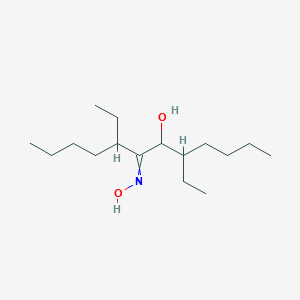
5,8-diéthyl-7-hydroxyiminododécan-6-ol
- Cliquez sur DEMANDE RAPIDE pour recevoir un devis de notre équipe d'experts.
- Avec des produits de qualité à un prix COMPÉTITIF, vous pouvez vous concentrer davantage sur votre recherche.
Vue d'ensemble
Description
5,8-Diethyl-7-hydroxydodecan-6-one oxime is a chemical compound with the molecular formula C16H33NO2. It is known for its use as a cationic extractant in the separation of metals, particularly in the recovery of indium and gallium from zinc refinery residues . The compound is characterized by its white to off-white solid form and has a melting point of 46-48°C .
Applications De Recherche Scientifique
5,8-Diethyl-7-hydroxydodecan-6-one oxime has several scientific research applications:
Chemistry: Used as a cationic extractant for the separation of metals, particularly indium and gallium.
Medicine: Research into its potential use in drug delivery systems due to its ability to form complexes with metal ions.
Industry: Widely used in the extraction and recovery of valuable metals from industrial residues.
Méthodes De Préparation
Synthetic Routes and Reaction Conditions
The synthesis of 5,8-Diethyl-7-hydroxydodecan-6-one oxime typically involves the reaction of 5,8-Diethyl-7-hydroxy-6-dodecanone with hydroxylamine. The reaction is carried out under controlled conditions to ensure the formation of the oxime group. The reaction conditions often include the use of solvents such as chloroform or ethyl acetate, which slightly dissolve the compound .
Industrial Production Methods
Industrial production of 5,8-Diethyl-7-hydroxydodecan-6-one oxime involves large-scale synthesis using similar reaction conditions as in laboratory settings. The compound is often produced in concentrations of 40-50% in kerosene for solvent extraction of metal ions .
Analyse Des Réactions Chimiques
Types of Reactions
5,8-Diethyl-7-hydroxydodecan-6-one oxime undergoes various chemical reactions, including:
Oxidation: The compound can be oxidized under specific conditions to form corresponding oxides.
Reduction: Reduction reactions can convert the oxime group back to the original ketone.
Substitution: The oxime group can participate in substitution reactions, where it is replaced by other functional groups.
Common Reagents and Conditions
Oxidation: Common oxidizing agents include potassium permanganate and hydrogen peroxide.
Reduction: Reducing agents such as sodium borohydride or lithium aluminum hydride are used.
Substitution: Various nucleophiles can be used for substitution reactions, depending on the desired product.
Major Products Formed
Oxidation: Corresponding oxides of the compound.
Reduction: 5,8-Diethyl-7-hydroxy-6-dodecanone.
Substitution: Various substituted derivatives depending on the nucleophile used.
Mécanisme D'action
The mechanism of action of 5,8-Diethyl-7-hydroxydodecan-6-one oxime involves its ability to form complexes with metal ions. The oxime group reacts with metal ions to form stable complexes, which can then be separated from the solution. This property makes it an effective extractant in metal recovery processes .
Comparaison Avec Des Composés Similaires
Similar Compounds
5,8-Diethyl-7-hydroxy-6-dodecanone: The precursor to the oxime compound.
5,8-Diethyl-7-hydroxydodecane-6-one oxime: Another name for the same compound.
6-Dodecanone, 5,8-diethyl-7-hydroxy-, oxime: A similar compound with slight variations in structure.
Uniqueness
5,8-Diethyl-7-hydroxydodecan-6-one oxime is unique due to its high efficiency in forming stable complexes with metal ions, making it particularly useful in the extraction and recovery of metals from industrial residues. Its ability to operate effectively in kerosene solutions further enhances its industrial applicability .
Activité Biologique
5,8-Diethyl-7-hydroxydodecan-6-one oxime, also known as LIX 63, is a compound that has garnered attention due to its potential applications in various fields, particularly in metal extraction and environmental remediation. This article explores its biological activity, including mechanisms of action, effects on different organisms, and relevant case studies.
- Molecular Formula : C14H27NO
- Molecular Weight : 227.38 g/mol
- Structure : The compound features a hydroxyl group and an oxime functional group, contributing to its reactivity and biological interactions.
The biological activity of LIX 63 primarily revolves around its role as a chelating agent. It interacts with metal ions, facilitating their extraction from aqueous solutions. This property is critical for applications in hydrometallurgy and environmental science.
Key Mechanisms:
- Metal Ion Complexation : LIX 63 forms stable complexes with various metal ions, enhancing their solubility and transportability in solution.
- Selective Extraction : The compound exhibits selective extraction capabilities for specific metals such as nickel (Ni) and cobalt (Co), making it valuable in recycling processes from spent solutions.
Toxicity Studies
Research indicates that LIX 63 has varying degrees of toxicity depending on the concentration and exposure duration. Studies have shown that:
- Aquatic Organisms : Exposure to LIX 63 can lead to toxic effects in aquatic species, including embryotoxicity in fish models such as Oryzias latipes (Japanese medaka) . Observed effects include vascular hemorrhage and developmental abnormalities.
- Invertebrates : The compound has been tested on Hydra attenuata, where it was compared with other polychlorinated compounds to assess its toxicological profile .
Case Studies
- Nickel Extraction from Electroplating Solutions :
- Environmental Remediation :
Comparative Analysis of Biological Activity
Propriétés
Numéro CAS |
6873-77-4 |
---|---|
Formule moléculaire |
C16H33NO2 |
Poids moléculaire |
271.44 g/mol |
Nom IUPAC |
(7E)-5,8-diethyl-7-hydroxyiminododecan-6-ol |
InChI |
InChI=1S/C16H33NO2/c1-5-9-11-13(7-3)15(17-19)16(18)14(8-4)12-10-6-2/h13-14,16,18-19H,5-12H2,1-4H3/b17-15+ |
Clé InChI |
SLCANKHLTWZHRV-BMRADRMJSA-N |
SMILES |
CCCCC(CC)C(C(=NO)C(CC)CCCC)O |
SMILES isomérique |
CCCCC(CC)C(/C(=N/O)/C(CC)CCCC)O |
SMILES canonique |
CCCCC(CC)C(C(=NO)C(CC)CCCC)O |
Key on ui other cas no. |
6873-77-4 |
Description physique |
Liquid |
Synonymes |
5,8-Diethyl-7-hydroxy-6-dodecanonoxime; 5,8-Diethyl-7-hydroxydodecan-6-oxime; 5,8-Diethyl-7-hydroxydodecane-6-oxime; LIX 63 |
Origine du produit |
United States |
Retrosynthesis Analysis
AI-Powered Synthesis Planning: Our tool employs the Template_relevance Pistachio, Template_relevance Bkms_metabolic, Template_relevance Pistachio_ringbreaker, Template_relevance Reaxys, Template_relevance Reaxys_biocatalysis model, leveraging a vast database of chemical reactions to predict feasible synthetic routes.
One-Step Synthesis Focus: Specifically designed for one-step synthesis, it provides concise and direct routes for your target compounds, streamlining the synthesis process.
Accurate Predictions: Utilizing the extensive PISTACHIO, BKMS_METABOLIC, PISTACHIO_RINGBREAKER, REAXYS, REAXYS_BIOCATALYSIS database, our tool offers high-accuracy predictions, reflecting the latest in chemical research and data.
Strategy Settings
Precursor scoring | Relevance Heuristic |
---|---|
Min. plausibility | 0.01 |
Model | Template_relevance |
Template Set | Pistachio/Bkms_metabolic/Pistachio_ringbreaker/Reaxys/Reaxys_biocatalysis |
Top-N result to add to graph | 6 |
Feasible Synthetic Routes
Q1: How does LIX 63 interact with metal ions and what factors influence its extraction efficiency?
A1: LIX 63 extracts metal ions through a chelation mechanism. The oxygen atom of the hydroxyl group and the nitrogen atom of the oxime group coordinate to the metal ion, forming a stable complex. [, ] Solvent effects play a significant role in LIX 63's extraction efficiency. The distribution constant (KDR) of LIX 63 between the organic and aqueous phases increases with solvent polarity. [] For instance, chloroform exhibits a higher KDR than n-heptane. This difference arises from the solvent's ability to stabilize the metal-LIX 63 complex. Additionally, factors like pH, metal ion concentration, and the presence of other competing ions can influence the extraction process.
Q2: Can you elaborate on the kinetics of uranium extraction using LIX 63 and how it is affected by the solvent?
A2: The extraction of uranium (VI) by LIX 63 (HR) follows a well-defined kinetic mechanism. [] The reaction is first-order with respect to uranium concentration and second-order with respect to LIX 63 concentration. Conversely, it demonstrates an inverse first-order dependence on hydrogen ion concentration. The rate-determining step is thought to be the reaction of the UO2R+ intermediate with a second LIX 63 molecule in the aqueous phase to form the final UO2R2 complex. Interestingly, the apparent rate constant shows an inverse relationship with the square of the KDR value. This finding supports the proposed mechanism, suggesting that solvents with higher KDR values, and thus a greater affinity for LIX 63, lead to lower concentrations of the reactive species in the aqueous phase, ultimately slowing down the extraction rate.
Q3: How selective is LIX 63 for different metal ions and what are the implications for separation processes?
A3: While LIX 63 effectively extracts uranium, its selectivity is crucial for separating target metals from complex mixtures. Research indicates that LIX 63 can separate molybdenum(VI) from tungsten(VI) under specific conditions. [] At a pH around 1, molybdenum(VI) is preferentially extracted with a high separation factor. This selectivity stems from the difference in extraction rates between the two metals. Additionally, LIX 63 can separate molybdenum(VI) from other metal ions like Fe(III), Co(II), Ni(II), Zn(II), and Cu(II), further highlighting its potential in metal separation and purification processes.
Avertissement et informations sur les produits de recherche in vitro
Veuillez noter que tous les articles et informations sur les produits présentés sur BenchChem sont destinés uniquement à des fins informatives. Les produits disponibles à l'achat sur BenchChem sont spécifiquement conçus pour des études in vitro, qui sont réalisées en dehors des organismes vivants. Les études in vitro, dérivées du terme latin "in verre", impliquent des expériences réalisées dans des environnements de laboratoire contrôlés à l'aide de cellules ou de tissus. Il est important de noter que ces produits ne sont pas classés comme médicaments et n'ont pas reçu l'approbation de la FDA pour la prévention, le traitement ou la guérison de toute condition médicale, affection ou maladie. Nous devons souligner que toute forme d'introduction corporelle de ces produits chez les humains ou les animaux est strictement interdite par la loi. Il est essentiel de respecter ces directives pour assurer la conformité aux normes légales et éthiques en matière de recherche et d'expérimentation.