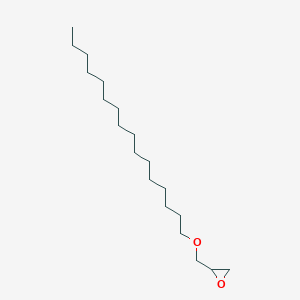
Éther de cétyle et de glycidyle
Vue d'ensemble
Description
Cetyl glycidyl ether, also known as 2-(hexadecyloxy)methyl)oxirane, is an organic compound with the molecular formula C19H38O2. It is a type of glycidyl ether, which is characterized by the presence of an epoxide group attached to an alkyl chain. Cetyl glycidyl ether is commonly used in various industrial applications due to its unique chemical properties.
Applications De Recherche Scientifique
Chemistry
Cetyl glycidyl ether is used as a reactive intermediate in the synthesis of various chemical compounds. Its epoxide group makes it a valuable building block for the production of polymers, resins, and surfactants.
Biology
In biological research, cetyl glycidyl ether is used as a cross-linking agent for proteins and other biomolecules. Its ability to form stable covalent bonds with nucleophiles makes it useful in the study of protein-protein interactions and enzyme mechanisms.
Medicine
Cetyl glycidyl ether is explored for its potential use in drug delivery systems. Its ability to form stable conjugates with therapeutic agents can enhance the stability and bioavailability of drugs.
Industry
In industrial applications, cetyl glycidyl ether is used as a surfactant and emulsifier in the formulation of cosmetics, personal care products, and lubricants. Its ability to reduce surface tension and improve the stability of emulsions makes it a valuable ingredient in these products.
Mécanisme D'action
Target of Action
Cetyl glycidyl ether, also known as glycidyl hexadecyl ether, is a chemical compound with the molecular formula C19H38O2 Glycidyl ethers, in general, are known to interact with various biological and chemical entities due to the high reactivity of their oxirane group .
Mode of Action
Glycidyl ethers are known for their reactivity, primarily due to the presence of an epoxide group . This group can undergo reactions with a variety of nucleophiles, leading to the opening of the epoxide ring and formation of new bonds . This property allows glycidyl ethers to participate in a wide range of chemical transformations.
Biochemical Pathways
Glycidyl ethers are known to be involved in the synthesis of glyceryl ethers . These compounds can be naturally isolated from shark liver oil and the skin of animals such as stingrays and chimeras and exhibit potential anti-fouling activity . The synthetic approach developed includes two distinct methods of preparation .
Pharmacokinetics
Information on the ADME (Absorption, Distribution, Metabolism, and Excretion) properties of cetyl glycidyl ether is limited. The physicochemical properties of the compound, such as its molecular weight and structure, suggest that it may have significant lipophilicity This could potentially influence its absorption and distribution within the body
Result of Action
Glycidyl ethers, in general, are known for their ability to react with a variety of substrates due to the presence of an epoxide group . This reactivity can lead to various chemical transformations, potentially resulting in the formation of new compounds with diverse properties.
Action Environment
The action of cetyl glycidyl ether can be influenced by various environmental factors. For instance, the hydrolysis of hydrophobic glycidyl ethers in pressurized water media can lead to the formation of the corresponding glyceryl ethers in good to excellent selectivity within several minutes without a catalyst . This suggests that the reaction environment can significantly influence the compound’s action, efficacy, and stability.
Analyse Biochimique
Biochemical Properties
For instance, glycidyl ethers of quercetin have been synthesized and their interactions with various groups have been studied . It’s plausible that Cetyl glycidyl ether may have similar interactions, but specific enzymes, proteins, or other biomolecules it interacts with are not currently known.
Cellular Effects
The specific cellular effects of Cetyl glycidyl ether are not well-documented. Glycidyl ethers have been shown to have certain effects on cells. For example, they have been used in the creation of new polymeric materials with nonlinear optical (NLO) properties, which have applications in photonics and optoelectronics
Molecular Mechanism
The molecular mechanism of action of Cetyl glycidyl ether is not well-documented. Glycidyl ethers have been shown to undergo certain reactions. For instance, glycidation of certain hydroxy groups has been observed in the synthesis of glycidyl ethers of quercetin . It’s plausible that Cetyl glycidyl ether may undergo similar reactions, but the specific binding interactions with biomolecules, enzyme inhibition or activation, and changes in gene expression are not currently known.
Temporal Effects in Laboratory Settings
Glycidyl ethers have been used in the synthesis of new polymeric materials, suggesting they have some degree of stability .
Dosage Effects in Animal Models
Animal models have played a critical role in exploring and describing disease pathophysiology and in testing new therapeutic agents .
Metabolic Pathways
The metabolic network of a living cell is highly intricate and involves complex interactions between various pathways .
Transport and Distribution
Glycidyl ethers have been used in the creation of new polymeric materials, suggesting they can be incorporated into a polymeric material as dopants or structural elements .
Subcellular Localization
Rna localization is essential for regulating spatial translation, where RNAs are trafficked to their target locations via various biological mechanisms .
Méthodes De Préparation
Synthetic Routes and Reaction Conditions
Cetyl glycidyl ether can be synthesized through the reaction of cetyl alcohol with epichlorohydrin in the presence of a base, such as sodium hydroxide. The reaction typically involves the following steps:
Formation of the alkoxide ion: Cetyl alcohol is reacted with sodium hydroxide to form the cetyl alkoxide ion.
Nucleophilic substitution: The cetyl alkoxide ion then reacts with epichlorohydrin, resulting in the formation of cetyl glycidyl ether.
The reaction conditions generally include a temperature range of 50-70°C and a reaction time of several hours to ensure complete conversion.
Industrial Production Methods
In industrial settings, cetyl glycidyl ether is produced using similar synthetic routes but on a larger scale. The process involves continuous flow reactors to maintain consistent reaction conditions and optimize yield. The use of catalysts, such as quaternary ammonium salts, can enhance the reaction rate and selectivity.
Analyse Des Réactions Chimiques
Types of Reactions
Cetyl glycidyl ether undergoes various chemical reactions, including:
Epoxide ring-opening reactions: The epoxide group in cetyl glycidyl ether is highly reactive and can undergo ring-opening reactions with nucleophiles such as amines, alcohols, and thiols.
Oxidation and reduction: The compound can be oxidized to form corresponding glycidyl esters or reduced to form alcohols.
Substitution reactions: The epoxide group can be substituted with other functional groups under appropriate conditions.
Common Reagents and Conditions
Nucleophiles: Amines, alcohols, thiols
Oxidizing agents: Hydrogen peroxide, peracids
Reducing agents: Lithium aluminum hydride, sodium borohydride
Major Products Formed
Epoxide ring-opening: Formation of glycidyl amines, glycidyl alcohols, and glycidyl thiols
Oxidation: Formation of glycidyl esters
Reduction: Formation of cetyl alcohol derivatives
Comparaison Avec Des Composés Similaires
Similar Compounds
Glycidyl ethers: Other glycidyl ethers, such as ethylene glycol diglycidyl ether and propylene glycol diglycidyl ether, share similar chemical properties with cetyl glycidyl ether.
Epoxides: Compounds like epichlorohydrin and glycidol also contain epoxide groups and exhibit similar reactivity.
Uniqueness
Cetyl glycidyl ether is unique due to its long alkyl chain, which imparts hydrophobic properties and enhances its surfactant and emulsifying capabilities. This makes it particularly useful in applications where both reactivity and hydrophobicity are desired.
Conclusion
Cetyl glycidyl ether is a versatile compound with a wide range of applications in chemistry, biology, medicine, and industry Its unique chemical properties, particularly the reactivity of its epoxide group, make it a valuable building block for various synthetic and industrial processes
Propriétés
IUPAC Name |
2-(hexadecoxymethyl)oxirane | |
---|---|---|
Source | PubChem | |
URL | https://pubchem.ncbi.nlm.nih.gov | |
Description | Data deposited in or computed by PubChem | |
InChI |
InChI=1S/C19H38O2/c1-2-3-4-5-6-7-8-9-10-11-12-13-14-15-16-20-17-19-18-21-19/h19H,2-18H2,1H3 | |
Source | PubChem | |
URL | https://pubchem.ncbi.nlm.nih.gov | |
Description | Data deposited in or computed by PubChem | |
InChI Key |
YZUMRMCHAJVDRT-UHFFFAOYSA-N | |
Source | PubChem | |
URL | https://pubchem.ncbi.nlm.nih.gov | |
Description | Data deposited in or computed by PubChem | |
Canonical SMILES |
CCCCCCCCCCCCCCCCOCC1CO1 | |
Source | PubChem | |
URL | https://pubchem.ncbi.nlm.nih.gov | |
Description | Data deposited in or computed by PubChem | |
Molecular Formula |
C19H38O2 | |
Source | PubChem | |
URL | https://pubchem.ncbi.nlm.nih.gov | |
Description | Data deposited in or computed by PubChem | |
DSSTOX Substance ID |
DTXSID50880874 | |
Record name | Cetyl glycidyl ether | |
Source | EPA DSSTox | |
URL | https://comptox.epa.gov/dashboard/DTXSID50880874 | |
Description | DSSTox provides a high quality public chemistry resource for supporting improved predictive toxicology. | |
Molecular Weight |
298.5 g/mol | |
Source | PubChem | |
URL | https://pubchem.ncbi.nlm.nih.gov | |
Description | Data deposited in or computed by PubChem | |
CAS No. |
15965-99-8 | |
Record name | Hexadecyl glycidyl ether | |
Source | CAS Common Chemistry | |
URL | https://commonchemistry.cas.org/detail?cas_rn=15965-99-8 | |
Description | CAS Common Chemistry is an open community resource for accessing chemical information. Nearly 500,000 chemical substances from CAS REGISTRY cover areas of community interest, including common and frequently regulated chemicals, and those relevant to high school and undergraduate chemistry classes. This chemical information, curated by our expert scientists, is provided in alignment with our mission as a division of the American Chemical Society. | |
Explanation | The data from CAS Common Chemistry is provided under a CC-BY-NC 4.0 license, unless otherwise stated. | |
Record name | Cetyl glycidyl ether | |
Source | ChemIDplus | |
URL | https://pubchem.ncbi.nlm.nih.gov/substance/?source=chemidplus&sourceid=0015965998 | |
Description | ChemIDplus is a free, web search system that provides access to the structure and nomenclature authority files used for the identification of chemical substances cited in National Library of Medicine (NLM) databases, including the TOXNET system. | |
Record name | Oxirane, 2-[(hexadecyloxy)methyl]- | |
Source | EPA Chemicals under the TSCA | |
URL | https://www.epa.gov/chemicals-under-tsca | |
Description | EPA Chemicals under the Toxic Substances Control Act (TSCA) collection contains information on chemicals and their regulations under TSCA, including non-confidential content from the TSCA Chemical Substance Inventory and Chemical Data Reporting. | |
Record name | Cetyl glycidyl ether | |
Source | EPA DSSTox | |
URL | https://comptox.epa.gov/dashboard/DTXSID50880874 | |
Description | DSSTox provides a high quality public chemistry resource for supporting improved predictive toxicology. | |
Record name | [(hexadecyloxy)methyl]oxirane | |
Source | European Chemicals Agency (ECHA) | |
URL | https://echa.europa.eu/substance-information/-/substanceinfo/100.036.442 | |
Description | The European Chemicals Agency (ECHA) is an agency of the European Union which is the driving force among regulatory authorities in implementing the EU's groundbreaking chemicals legislation for the benefit of human health and the environment as well as for innovation and competitiveness. | |
Explanation | Use of the information, documents and data from the ECHA website is subject to the terms and conditions of this Legal Notice, and subject to other binding limitations provided for under applicable law, the information, documents and data made available on the ECHA website may be reproduced, distributed and/or used, totally or in part, for non-commercial purposes provided that ECHA is acknowledged as the source: "Source: European Chemicals Agency, http://echa.europa.eu/". Such acknowledgement must be included in each copy of the material. ECHA permits and encourages organisations and individuals to create links to the ECHA website under the following cumulative conditions: Links can only be made to webpages that provide a link to the Legal Notice page. | |
Record name | CETYL GLYCIDYL ETHER | |
Source | FDA Global Substance Registration System (GSRS) | |
URL | https://gsrs.ncats.nih.gov/ginas/app/beta/substances/T11C352FYU | |
Description | The FDA Global Substance Registration System (GSRS) enables the efficient and accurate exchange of information on what substances are in regulated products. Instead of relying on names, which vary across regulatory domains, countries, and regions, the GSRS knowledge base makes it possible for substances to be defined by standardized, scientific descriptions. | |
Explanation | Unless otherwise noted, the contents of the FDA website (www.fda.gov), both text and graphics, are not copyrighted. They are in the public domain and may be republished, reprinted and otherwise used freely by anyone without the need to obtain permission from FDA. Credit to the U.S. Food and Drug Administration as the source is appreciated but not required. | |
Synthesis routes and methods
Procedure details
Retrosynthesis Analysis
AI-Powered Synthesis Planning: Our tool employs the Template_relevance Pistachio, Template_relevance Bkms_metabolic, Template_relevance Pistachio_ringbreaker, Template_relevance Reaxys, Template_relevance Reaxys_biocatalysis model, leveraging a vast database of chemical reactions to predict feasible synthetic routes.
One-Step Synthesis Focus: Specifically designed for one-step synthesis, it provides concise and direct routes for your target compounds, streamlining the synthesis process.
Accurate Predictions: Utilizing the extensive PISTACHIO, BKMS_METABOLIC, PISTACHIO_RINGBREAKER, REAXYS, REAXYS_BIOCATALYSIS database, our tool offers high-accuracy predictions, reflecting the latest in chemical research and data.
Strategy Settings
Precursor scoring | Relevance Heuristic |
---|---|
Min. plausibility | 0.01 |
Model | Template_relevance |
Template Set | Pistachio/Bkms_metabolic/Pistachio_ringbreaker/Reaxys/Reaxys_biocatalysis |
Top-N result to add to graph | 6 |
Feasible Synthetic Routes
Q1: What are the main applications of Glycidyl hexadecyl ether in material science?
A1: Glycidyl hexadecyl ether (also known as Cetyl glycidyl ether) is primarily used as a hydrophobic modifier for various materials. For instance, it can be grafted onto polysaccharides like guar, hydroxyethyl guar, hyaluronic acid, and bacterial cellulose to alter their properties. [, ] This modification can lead to the formation of amphiphilic systems, potentially influencing the material's viscosity, self-assembly behavior, and interaction with aqueous environments. [] Additionally, it has been used in the development of antimicrobial coatings for surgical sutures, contributing to the long-term release of silver nanoparticles. []
Q2: How does Glycidyl hexadecyl ether contribute to the development of antibacterial materials?
A2: Glycidyl hexadecyl ether can be used as an active agent to chemically modify materials like bacterial cellulose, imparting antibacterial properties. [] This is achieved by covalently attaching the molecule to the hydroxyl groups of the cellulose structure. While the exact mechanism of action is not fully elucidated in the provided research, this modification has shown a significant reduction in bacterial adhesion and growth for both Staphylococcus aureus and Escherichia coli. []
Q3: Can you explain the role of Glycidyl hexadecyl ether in the synthesis of lipophilic polyamines and their potential applications?
A3: Glycidyl hexadecyl ether serves as a crucial building block in synthesizing lipophilic polyamines. [] The process involves reacting it with polyamines like norspermine or triethylenetetramine, utilizing the reactivity of the epoxide ring. This reaction results in the incorporation of a lipophilic hexadecyl chain into the polyamine structure. These modified polyamines have shown potential as antitumor agents, as their lipophilic nature might enhance their cellular uptake and interaction with biological targets. []
Q4: How does the incorporation of Glycidyl hexadecyl ether affect the properties of epoxy-based dielectric materials?
A4: Research suggests that incorporating Glycidyl hexadecyl ether as a functional network modifier (FNM) in epoxy-based networks can significantly alter their dielectric properties. [] Specifically, it leads to a decrease in the glass transition temperature (Tg), a reduction in permittivity, and lower dielectric losses. [] Additionally, it enhances the DC conductivity and AC breakdown strength of the material. [] These changes are attributed to the specific chemical interactions of the Glycidyl hexadecyl ether within the epoxy network, influencing the material's overall structure and electrical behavior. []
Q5: Are there any studies exploring the use of Glycidyl hexadecyl ether in drug delivery systems?
A5: Yes, Glycidyl hexadecyl ether has been investigated in the context of gene delivery. [] It was used to synthesize PEI lipids, which were then incorporated into cationic liposomes and PLGA nanoparticles. [] These systems, designed for delivering plasmid DNA, demonstrated lower toxicity and enhanced transfection efficacy compared to traditional high molecular weight PEI-based systems. [] This suggests the potential of Glycidyl hexadecyl ether-derived components in improving the efficiency and safety of gene delivery platforms.
Avertissement et informations sur les produits de recherche in vitro
Veuillez noter que tous les articles et informations sur les produits présentés sur BenchChem sont destinés uniquement à des fins informatives. Les produits disponibles à l'achat sur BenchChem sont spécifiquement conçus pour des études in vitro, qui sont réalisées en dehors des organismes vivants. Les études in vitro, dérivées du terme latin "in verre", impliquent des expériences réalisées dans des environnements de laboratoire contrôlés à l'aide de cellules ou de tissus. Il est important de noter que ces produits ne sont pas classés comme médicaments et n'ont pas reçu l'approbation de la FDA pour la prévention, le traitement ou la guérison de toute condition médicale, affection ou maladie. Nous devons souligner que toute forme d'introduction corporelle de ces produits chez les humains ou les animaux est strictement interdite par la loi. Il est essentiel de respecter ces directives pour assurer la conformité aux normes légales et éthiques en matière de recherche et d'expérimentation.