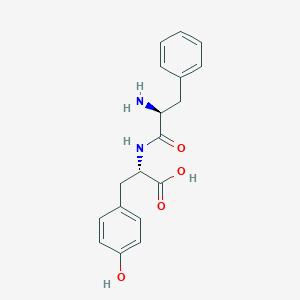
Phénylalanine-tyrosine
Vue d'ensemble
Description
La phénylalan yltyrosine est un dipeptide composé des acides aminés phénylalanine et tyrosine. C'est un produit de dégradation incomplet de la digestion des protéines ou du catabolisme des protéines. Les dipeptides sont des composés organiques contenant une séquence d'exactement deux α-acides aminés liés par une liaison peptidique .
Applications De Recherche Scientifique
Phenylalanyltyrosine has a wide range of applications in scientific research:
Chemistry: Used as a building block for the synthesis of more complex molecules.
Biology: Studied for its role in protein synthesis and metabolism.
Medicine: Investigated for its potential therapeutic effects and as a biomarker for certain diseases.
Mécanisme D'action
Phenylalanyltyrosine exerts its effects through various molecular pathways:
Protein Synthesis: It is incorporated into proteins during translation, facilitated by aminoacyl-tRNA synthetases.
Metabolic Pathways: It participates in the phenylalanine and tyrosine metabolic pathways, leading to the production of important metabolites such as dopamine and melanin.
Safety and Hazards
Analyse Biochimique
Biochemical Properties
Phenylalanyltyrosine interacts with various enzymes, proteins, and other biomolecules. The metabolic pathway responsible for the biosynthesis of aromatic amino acids, such as phenylalanine and tyrosine, is a major enzyme network in nature . This pathway interfaces with carbohydrate metabolism at the reaction catalyzed by 3-deoxy-D-arabino-heptulosonate 7-phosphate (DAHP) synthase .
Cellular Effects
Phenylalanyltyrosine has significant effects on various types of cells and cellular processes. High levels of this amino acid impair mitochondrial bioenergetics, provoke changes in oxidative and inflammatory status, and induce apoptosis .
Molecular Mechanism
Phenylalanyltyrosine exerts its effects at the molecular level through various mechanisms. For instance, inherent promiscuity of bacterial translation is demonstrated by mass spectrometric quantification of the translational incorporation of ring-substituted phenylalanines and tyrosines bearing fluoro-, hydroxyl-, methyl-, chloro- and nitro-groups in an E. coli-derived cell-free system .
Dosage Effects in Animal Models
They allow scientists to predict outcomes and understand complex biological processes .
Metabolic Pathways
Phenylalanyltyrosine is involved in the metabolic pathway responsible for the biosynthesis of aromatic amino acids . This pathway interfaces with carbohydrate metabolism at the reaction catalyzed by DAHP synthase .
Transport and Distribution
It is known that separate biochemical pathways of aromatic biosynthesis may exist in the spatially separated microenvironments of the plastid and cytosolic compartments .
Subcellular Localization
It is known that separate biochemical pathways of aromatic biosynthesis may exist in the spatially separated microenvironments of the plastid and cytosolic compartments .
Méthodes De Préparation
Voies de synthèse et conditions de réaction
La phénylalan yltyrosine peut être synthétisée par condensation de la phénylalanine et de la tyrosine. Cette réaction implique généralement l'utilisation d'un réactif de couplage tel que le dicyclohexylcarbodiimide (DCC) en présence d'une base comme la triéthylamine. La réaction est effectuée dans un solvant organique tel que le diméthylformamide (DMF) à température ambiante .
Méthodes de production industrielle
Dans les milieux industriels, la production de phénylalan yltyrosine peut impliquer l'utilisation de méthodes enzymatiques. Des enzymes telles que les aminoacyl-tRNA synthétases peuvent être utilisées pour catalyser la formation du dipeptide à partir de ses acides aminés constitutifs. Cette méthode offre une grande spécificité et une grande efficacité .
Analyse Des Réactions Chimiques
Types de réactions
La phénylalan yltyrosine subit diverses réactions chimiques, notamment :
Oxydation : Le groupe hydroxyle phénolique de la tyrosine peut être oxydé pour former des quinones.
Réduction : Les groupes nitro sur les dérivés de la phénylalanine substitués peuvent être réduits en amines.
Substitution : Les dérivés halogénés de la phénylalanine peuvent subir des réactions de substitution nucléophile.
Réactifs et conditions courants
Oxydation : Des réactifs tels que le peroxyde d'hydrogène ou le permanganate de potassium sont couramment utilisés.
Réduction : Hydrogénation catalytique ou utilisation d'agents réducteurs comme le borohydrure de sodium.
Substitution : Des nucléophiles tels que l'azoture de sodium ou les thiols peuvent être utilisés en conditions basiques.
Principaux produits formés
Oxydation : Quinones et autres dérivés oxydés.
Réduction : Amines et dérivés réduits.
Substitution : Dérivés de la phénylalanine substitués.
Applications de la recherche scientifique
La phénylalan yltyrosine a un large éventail d'applications dans la recherche scientifique :
Chimie : Utilisée comme élément constitutif pour la synthèse de molécules plus complexes.
Biologie : Étudiée pour son rôle dans la synthèse et le métabolisme des protéines.
Médecine : Étudiée pour ses effets thérapeutiques potentiels et comme biomarqueur de certaines maladies.
Mécanisme d'action
La phénylalan yltyrosine exerce ses effets par le biais de diverses voies moléculaires :
Synthèse des protéines : Elle est incorporée aux protéines pendant la traduction, facilitée par les aminoacyl-tRNA synthétases.
Voies métaboliques : Elle participe aux voies métaboliques de la phénylalanine et de la tyrosine, conduisant à la production de métabolites importants tels que la dopamine et la mélanine.
Comparaison Avec Des Composés Similaires
La phénylalan yltyrosine est similaire à d'autres dipeptides composés d'acides aminés aromatiques, tels que :
Phénylalan ylglycine : Composé de phénylalanine et de glycine.
Tyrosylglycine : Composé de tyrosine et de glycine.
Phénylalan ylleucine : Composé de phénylalanine et de leucine.
Unicité
La phénylalan yltyrosine est unique en raison de la présence à la fois de phénylalanine et de tyrosine, ce qui lui permet de participer à un large éventail de réactions et de voies biochimiques. Cette double composition améliore également son potentiel en tant qu'agent thérapeutique et outil de recherche .
Propriétés
IUPAC Name |
(2S)-2-[[(2S)-2-amino-3-phenylpropanoyl]amino]-3-(4-hydroxyphenyl)propanoic acid | |
---|---|---|
Source | PubChem | |
URL | https://pubchem.ncbi.nlm.nih.gov | |
Description | Data deposited in or computed by PubChem | |
InChI |
InChI=1S/C18H20N2O4/c19-15(10-12-4-2-1-3-5-12)17(22)20-16(18(23)24)11-13-6-8-14(21)9-7-13/h1-9,15-16,21H,10-11,19H2,(H,20,22)(H,23,24)/t15-,16-/m0/s1 | |
Source | PubChem | |
URL | https://pubchem.ncbi.nlm.nih.gov | |
Description | Data deposited in or computed by PubChem | |
InChI Key |
FSXRLASFHBWESK-HOTGVXAUSA-N | |
Source | PubChem | |
URL | https://pubchem.ncbi.nlm.nih.gov | |
Description | Data deposited in or computed by PubChem | |
Canonical SMILES |
C1=CC=C(C=C1)CC(C(=O)NC(CC2=CC=C(C=C2)O)C(=O)O)N | |
Source | PubChem | |
URL | https://pubchem.ncbi.nlm.nih.gov | |
Description | Data deposited in or computed by PubChem | |
Isomeric SMILES |
C1=CC=C(C=C1)C[C@@H](C(=O)N[C@@H](CC2=CC=C(C=C2)O)C(=O)O)N | |
Source | PubChem | |
URL | https://pubchem.ncbi.nlm.nih.gov | |
Description | Data deposited in or computed by PubChem | |
Molecular Formula |
C18H20N2O4 | |
Source | PubChem | |
URL | https://pubchem.ncbi.nlm.nih.gov | |
Description | Data deposited in or computed by PubChem | |
DSSTOX Substance ID |
DTXSID30333656 | |
Record name | L-Phenylalanyl-L-tyrosine | |
Source | EPA DSSTox | |
URL | https://comptox.epa.gov/dashboard/DTXSID30333656 | |
Description | DSSTox provides a high quality public chemistry resource for supporting improved predictive toxicology. | |
Molecular Weight |
328.4 g/mol | |
Source | PubChem | |
URL | https://pubchem.ncbi.nlm.nih.gov | |
Description | Data deposited in or computed by PubChem | |
Physical Description |
Solid | |
Record name | Phenylalanyltyrosine | |
Source | Human Metabolome Database (HMDB) | |
URL | http://www.hmdb.ca/metabolites/HMDB0029007 | |
Description | The Human Metabolome Database (HMDB) is a freely available electronic database containing detailed information about small molecule metabolites found in the human body. | |
Explanation | HMDB is offered to the public as a freely available resource. Use and re-distribution of the data, in whole or in part, for commercial purposes requires explicit permission of the authors and explicit acknowledgment of the source material (HMDB) and the original publication (see the HMDB citing page). We ask that users who download significant portions of the database cite the HMDB paper in any resulting publications. | |
CAS No. |
17355-18-9 | |
Record name | L-Phenylalanyl-L-tyrosine | |
Source | EPA DSSTox | |
URL | https://comptox.epa.gov/dashboard/DTXSID30333656 | |
Description | DSSTox provides a high quality public chemistry resource for supporting improved predictive toxicology. | |
Record name | Phenylalanyltyrosine | |
Source | Human Metabolome Database (HMDB) | |
URL | http://www.hmdb.ca/metabolites/HMDB0029007 | |
Description | The Human Metabolome Database (HMDB) is a freely available electronic database containing detailed information about small molecule metabolites found in the human body. | |
Explanation | HMDB is offered to the public as a freely available resource. Use and re-distribution of the data, in whole or in part, for commercial purposes requires explicit permission of the authors and explicit acknowledgment of the source material (HMDB) and the original publication (see the HMDB citing page). We ask that users who download significant portions of the database cite the HMDB paper in any resulting publications. | |
Retrosynthesis Analysis
AI-Powered Synthesis Planning: Our tool employs the Template_relevance Pistachio, Template_relevance Bkms_metabolic, Template_relevance Pistachio_ringbreaker, Template_relevance Reaxys, Template_relevance Reaxys_biocatalysis model, leveraging a vast database of chemical reactions to predict feasible synthetic routes.
One-Step Synthesis Focus: Specifically designed for one-step synthesis, it provides concise and direct routes for your target compounds, streamlining the synthesis process.
Accurate Predictions: Utilizing the extensive PISTACHIO, BKMS_METABOLIC, PISTACHIO_RINGBREAKER, REAXYS, REAXYS_BIOCATALYSIS database, our tool offers high-accuracy predictions, reflecting the latest in chemical research and data.
Strategy Settings
Precursor scoring | Relevance Heuristic |
---|---|
Min. plausibility | 0.01 |
Model | Template_relevance |
Template Set | Pistachio/Bkms_metabolic/Pistachio_ringbreaker/Reaxys/Reaxys_biocatalysis |
Top-N result to add to graph | 6 |
Feasible Synthetic Routes
Q1: How does phenylalanyltyrosine interact with biological systems to exert its effects, particularly regarding blood pressure regulation?
A1: Current research suggests that phenylalanyltyrosine may interact with the renin-angiotensin-aldosterone system (RAAS), a key regulator of blood pressure. [] This system is responsible for maintaining blood pressure homeostasis, and its dysregulation is implicated in hypertension. While the precise mechanism of action remains under investigation, computational studies using molecular docking have been employed to explore phenylalanyltyrosine's potential as an inhibitor of key RAAS enzymes like angiotensin-converting enzyme (ACE) and as a blocker of angiotensin II type 1 receptors (AT1R). [] These enzymes and receptors play critical roles in the RAAS cascade, ultimately influencing blood vessel constriction and fluid balance. Inhibiting ACE or blocking AT1R could potentially lead to vasodilation and a decrease in blood pressure. Further experimental validation is needed to confirm these interactions and elucidate the specific mechanisms underlying phenylalanyltyrosine's effects on blood pressure.
Q2: What is known about the structural characteristics of phenylalanyltyrosine?
A2: While a detailed spectroscopic characterization is not available in the provided abstracts, we know that phenylalanyltyrosine (H-Phe-Tyr-OH) is a dipeptide consisting of L-phenylalanine and L-tyrosine linked by a peptide bond. [] The presence of a phenyl group in the structure appears crucial for its biological activity, specifically its ability to reduce cytochrome C. [] Further research, including techniques like nuclear magnetic resonance (NMR) and infrared (IR) spectroscopy, could provide a more comprehensive understanding of its structural features and how they relate to its activity.
Avertissement et informations sur les produits de recherche in vitro
Veuillez noter que tous les articles et informations sur les produits présentés sur BenchChem sont destinés uniquement à des fins informatives. Les produits disponibles à l'achat sur BenchChem sont spécifiquement conçus pour des études in vitro, qui sont réalisées en dehors des organismes vivants. Les études in vitro, dérivées du terme latin "in verre", impliquent des expériences réalisées dans des environnements de laboratoire contrôlés à l'aide de cellules ou de tissus. Il est important de noter que ces produits ne sont pas classés comme médicaments et n'ont pas reçu l'approbation de la FDA pour la prévention, le traitement ou la guérison de toute condition médicale, affection ou maladie. Nous devons souligner que toute forme d'introduction corporelle de ces produits chez les humains ou les animaux est strictement interdite par la loi. Il est essentiel de respecter ces directives pour assurer la conformité aux normes légales et éthiques en matière de recherche et d'expérimentation.