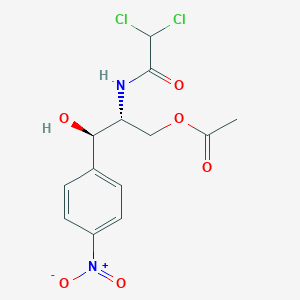
Chloramphenicol 3-acetate
Vue d'ensemble
Description
Chloramphenicol 3-acetate is a metabolite of Chloramphenicol . It is a broad-spectrum antibiotic that is effective against a variety of susceptible and serious bacterial infections . It is primarily bacteriostatic .
Synthesis Analysis
The initial biotransformation steps of Chloramphenicol include the oxidization at the C1-OH and C3-OH groups, the isomerization at C2, and the acetylation at C3-OH . A novel glucose-methanol-choline oxidoreductase responsible for the oxidization of the C3-OH group in Sphingomonas sp. and Caballeronia sp. has been identified .Molecular Structure Analysis
Chloramphenicol 3-acetate has a chemical formula of C13H14Cl2N2O6 . It is known that the C1 and C2 of chloramphenicol are chiral carbon atoms, and four stereoisomers exist .Chemical Reactions Analysis
Chloramphenicol can be stereoselectively transformed via microbial metabolism with R,R- (-)- CAP being subject to extensive metabolic transformation by an environmental bacterial strain . In contrast, S,S- (+) - CAP is not metabolized by this bacterial strain, possibly due to the lack of previous exposure to this isomer in the absence of historical selective pressure to evolve metabolic capacity .Physical And Chemical Properties Analysis
Chloramphenicol 3-acetate has an average weight of 365.16 and a monoisotopic weight of 364.0228916 . It has a chemical formula of C13H14Cl2N2O6 .Applications De Recherche Scientifique
Bioremediation
Chloramphenicol 3-acetate plays a significant role in bioremediation, particularly in the degradation of chloramphenicol residues in the environment. Microorganisms in activated sludge systems can biotransform chloramphenicol through oxidation, isomerization, and acetylation processes . This biotransformation is crucial for mitigating the environmental impact of chloramphenicol, which can disrupt ecosystems and contribute to the emergence of antibiotic resistance genes .
Environmental Monitoring
The presence of chloramphenicol 3-acetate in environmental waters is a concern due to its potential toxicity and contribution to antibiotic resistance. Advanced analytical methods, such as liquid chromatography coupled with mass spectrometry, are used to monitor its occurrence and concentrations in various water bodies, aiding in the assessment of environmental risks .
Medical Research
In medical research, chloramphenicol 3-acetate is utilized to study bacterial resistance mechanisms. It serves as a model compound to understand how bacteria metabolize antibiotics and develop resistance, which is critical for developing new strategies to combat antibiotic-resistant infections .
Veterinary Medicine
Although its use is restricted in food-producing animals, chloramphenicol 3-acetate is studied for its efficacy and safety in veterinary medicine. Research focuses on its therapeutic potential, pharmacokinetics, and possible adverse effects in non-food-producing animals .
Food Safety
Chloramphenicol 3-acetate is relevant in food safety research due to its potential presence in food of animal origin. Studies focus on developing sensitive detection methods to ensure that food products are free from chloramphenicol residues, which are harmful to human health .
Agriculture
Research in agriculture examines the impact of chloramphenicol 3-acetate on food-producing animals and the environment. It includes studying the compound’s presence in feed, its effects on animal health, and the potential for bioaccumulation in the food chain .
Antibiotic Removal Techniques
Chloramphenicol 3-acetate is used in studies exploring adsorptive removal techniques from water sources. Various adsorbents, such as biochar, activated carbon, and molecularly imprinted polymers, are evaluated for their efficiency in removing this antibiotic, which is crucial for water purification processes .
Pharmaceutical Wastewater Treatment
The compound’s role in the treatment of pharmaceutical wastewater is investigated to enhance the degradation of antibiotic contaminants. Research aims to identify microbial strains and enzymes that can effectively break down chloramphenicol 3-acetate, reducing its environmental footprint .
Mécanisme D'action
Target of Action
Chloramphenicol 3-acetate, also known as chloramphenicol acetate, primarily targets the bacterial ribosome . The compound binds to the 23S rRNA on the 50S subunit of the bacterial ribosome . This interaction inhibits the action of peptidyl transferase , an enzyme that plays a crucial role in protein synthesis .
Mode of Action
Chloramphenicol 3-acetate interacts with its targets by binding to the bacterial ribosome and blocking peptidyl transferase . This action inhibits protein synthesis, thereby stopping bacterial growth . The compound is lipid-soluble, which allows it to diffuse through the bacterial cell membrane .
Biochemical Pathways
The initial biotransformation steps of chloramphenicol include the oxidization at the C1-OH and C3-OH groups, the isomerization at C2, and the acetylation at C3-OH . Among these, the isomerization represents a newly discovered biotransformation pathway of chloramphenicol . A novel glucose-methanol-choline oxidoreductase has been identified as responsible for the oxidization of the C3-OH group in certain bacterial species .
Pharmacokinetics
The pharmacokinetics of chloramphenicol 3-acetate, including its Absorption, Distribution, Metabolism, and Excretion (ADME) properties, are crucial for understanding its bioavailability. Chloramphenicol is known to be lipid-soluble, which allows it to diffuse through the bacterial cell membrane . .
Result of Action
The primary result of chloramphenicol 3-acetate’s action is the inhibition of bacterial growth . By binding to the bacterial ribosome and blocking peptidyl transferase, the compound inhibits protein synthesis, effectively stopping the growth of the bacteria .
Action Environment
Environmental factors can influence the action, efficacy, and stability of chloramphenicol 3-acetate. For instance, the compound is prone to be released into environments, resulting in the disturbance of ecosystem stability and the emergence of antibiotic resistance genes . Microbes play a vital role in the decomposition of chloramphenicol in the environment, and the biotransformation processes are especially dependent on synergistic interactions and metabolite exchanges among microbes .
Safety and Hazards
Chloramphenicol 3-acetate may cause serious eye damage, is suspected of causing cancer, and is suspected of damaging fertility or the unborn child . It is advised to obtain special instructions before use, do not handle until all safety precautions have been read and understood, and to wear personal protective equipment/face protection .
Orientations Futures
The in-depth understanding of the Chloramphenicol biotransformation mechanisms and microbial interactions will not only guide the bioremediation of organic pollutants but also provide valuable knowledge for environmental microbiology and biotechnological exploitation . This study provides desirable strain and enzyme resources for enhanced bioremediation of Chloramphenicol-contaminated hotspot sites such as pharmaceutical wastewater and livestock and poultry wastewater .
Propriétés
IUPAC Name |
[(2R,3R)-2-[(2,2-dichloroacetyl)amino]-3-hydroxy-3-(4-nitrophenyl)propyl] acetate | |
---|---|---|
Source | PubChem | |
URL | https://pubchem.ncbi.nlm.nih.gov | |
Description | Data deposited in or computed by PubChem | |
InChI |
InChI=1S/C13H14Cl2N2O6/c1-7(18)23-6-10(16-13(20)12(14)15)11(19)8-2-4-9(5-3-8)17(21)22/h2-5,10-12,19H,6H2,1H3,(H,16,20)/t10-,11-/m1/s1 | |
Source | PubChem | |
URL | https://pubchem.ncbi.nlm.nih.gov | |
Description | Data deposited in or computed by PubChem | |
InChI Key |
VVOIFRARHIZCJD-GHMZBOCLSA-N | |
Source | PubChem | |
URL | https://pubchem.ncbi.nlm.nih.gov | |
Description | Data deposited in or computed by PubChem | |
Canonical SMILES |
CC(=O)OCC(C(C1=CC=C(C=C1)[N+](=O)[O-])O)NC(=O)C(Cl)Cl | |
Source | PubChem | |
URL | https://pubchem.ncbi.nlm.nih.gov | |
Description | Data deposited in or computed by PubChem | |
Isomeric SMILES |
CC(=O)OC[C@H]([C@@H](C1=CC=C(C=C1)[N+](=O)[O-])O)NC(=O)C(Cl)Cl | |
Source | PubChem | |
URL | https://pubchem.ncbi.nlm.nih.gov | |
Description | Data deposited in or computed by PubChem | |
Molecular Formula |
C13H14Cl2N2O6 | |
Source | PubChem | |
URL | https://pubchem.ncbi.nlm.nih.gov | |
Description | Data deposited in or computed by PubChem | |
DSSTOX Substance ID |
DTXSID30908222 | |
Record name | N-[3-(Acetyloxy)-1-hydroxy-1-(4-nitrophenyl)propan-2-yl]-2,2-dichloroethanimidic acid | |
Source | EPA DSSTox | |
URL | https://comptox.epa.gov/dashboard/DTXSID30908222 | |
Description | DSSTox provides a high quality public chemistry resource for supporting improved predictive toxicology. | |
Molecular Weight |
365.16 g/mol | |
Source | PubChem | |
URL | https://pubchem.ncbi.nlm.nih.gov | |
Description | Data deposited in or computed by PubChem | |
Product Name |
Chloramphenicol 3-acetate | |
CAS RN |
10318-16-8 | |
Record name | N-[(1R,2R)-1-[(Acetyloxy)methyl]-2-hydroxy-2-(4-nitrophenyl)ethyl]-2,2-dichloroacetamide | |
Source | CAS Common Chemistry | |
URL | https://commonchemistry.cas.org/detail?cas_rn=10318-16-8 | |
Description | CAS Common Chemistry is an open community resource for accessing chemical information. Nearly 500,000 chemical substances from CAS REGISTRY cover areas of community interest, including common and frequently regulated chemicals, and those relevant to high school and undergraduate chemistry classes. This chemical information, curated by our expert scientists, is provided in alignment with our mission as a division of the American Chemical Society. | |
Explanation | The data from CAS Common Chemistry is provided under a CC-BY-NC 4.0 license, unless otherwise stated. | |
Record name | Chloramphenicol 3-acetate | |
Source | ChemIDplus | |
URL | https://pubchem.ncbi.nlm.nih.gov/substance/?source=chemidplus&sourceid=0010318168 | |
Description | ChemIDplus is a free, web search system that provides access to the structure and nomenclature authority files used for the identification of chemical substances cited in National Library of Medicine (NLM) databases, including the TOXNET system. | |
Record name | N-[3-(Acetyloxy)-1-hydroxy-1-(4-nitrophenyl)propan-2-yl]-2,2-dichloroethanimidic acid | |
Source | EPA DSSTox | |
URL | https://comptox.epa.gov/dashboard/DTXSID30908222 | |
Description | DSSTox provides a high quality public chemistry resource for supporting improved predictive toxicology. | |
Retrosynthesis Analysis
AI-Powered Synthesis Planning: Our tool employs the Template_relevance Pistachio, Template_relevance Bkms_metabolic, Template_relevance Pistachio_ringbreaker, Template_relevance Reaxys, Template_relevance Reaxys_biocatalysis model, leveraging a vast database of chemical reactions to predict feasible synthetic routes.
One-Step Synthesis Focus: Specifically designed for one-step synthesis, it provides concise and direct routes for your target compounds, streamlining the synthesis process.
Accurate Predictions: Utilizing the extensive PISTACHIO, BKMS_METABOLIC, PISTACHIO_RINGBREAKER, REAXYS, REAXYS_BIOCATALYSIS database, our tool offers high-accuracy predictions, reflecting the latest in chemical research and data.
Strategy Settings
Precursor scoring | Relevance Heuristic |
---|---|
Min. plausibility | 0.01 |
Model | Template_relevance |
Template Set | Pistachio/Bkms_metabolic/Pistachio_ringbreaker/Reaxys/Reaxys_biocatalysis |
Top-N result to add to graph | 6 |
Feasible Synthetic Routes
Q & A
Q1: What is the mechanism behind chloramphenicol resistance in bacteria?
A: Many bacteria, including Staphylococcus aureus and Escherichia coli, develop resistance to chloramphenicol by producing an enzyme called chloramphenicol acetyltransferase (CAT). This enzyme adds an acetyl group to chloramphenicol, specifically at the 3-hydroxyl group, forming chloramphenicol 3-acetate. [, , ] This modification prevents chloramphenicol from binding to its target, the bacterial ribosome, thereby inactivating the antibiotic. [, ] Interestingly, chloramphenicol 3-acetate itself does not induce the production of CAT. []
Q2: Are there other mechanisms of chloramphenicol inactivation in bacteria?
A: Yes, besides acetylation, a novel chloramphenicol hydrolase enzyme (EstDL136) has been identified through metagenomic analysis. [, ] This enzyme hydrolyzes chloramphenicol, breaking it down into inactive compounds, one of which has been identified as p-nitrophenylserinol. [] Notably, this enzyme also inactivates florfenicol, another antibiotic, suggesting a broader substrate specificity. []
Q3: How is chloramphenicol acetyltransferase activity typically assayed?
A: A common method for detecting and quantifying CAT activity involves separating chloramphenicol 3-acetate from unreacted chloramphenicol using adsorption chromatography with a neutral alumina column. [] Following separation, chloramphenicol 3-acetate can be quantified either through colorimetric analysis after chemical derivatization or through a microbiological assay after enzymatic deacetylation. []
Q4: Does the production of chloramphenicol acetyltransferase always confer resistance?
A: Interestingly, the presence of CAT might not guarantee chloramphenicol resistance in all microorganisms. Studies on methanogens found that chloramphenicol 3-acetate still inhibited their growth. [] This suggests that the introduction of CAT-encoding genes may not be sufficient to confer resistance in these organisms. []
Q5: Is chloramphenicol 3-acetate found naturally?
A: Chloramphenicol 3-acetate is not solely a product of bacterial resistance mechanisms. Streptomyces griseus, a species known to naturally produce chloramphenicol, possesses chloramphenicol acetyltransferase primarily located on the surface of its spores. [, ] This enzyme specifically catalyzes the formation of chloramphenicol 3-acetate. [] Interestingly, these spores can even utilize chloramphenicol 3-acetate as a substrate for further modifications, adding different acyl groups to generate other chloramphenicol esters. []
Q6: Are there alternative methods to screen for novel enzymes involved in chloramphenicol resistance?
A: Function-driven metagenomic analysis has proven to be a valuable tool for discovering new enzymes involved in antibiotic resistance. [] This approach led to the identification of two novel esterases, EstDL26 and EstDL136, from a soil metagenomic library. [] These esterases can reactivate chloramphenicol from its acetylated forms by counteracting the action of CAT. [] This highlights the potential of metagenomics for uncovering novel resistance mechanisms and developing strategies to overcome them.
Avertissement et informations sur les produits de recherche in vitro
Veuillez noter que tous les articles et informations sur les produits présentés sur BenchChem sont destinés uniquement à des fins informatives. Les produits disponibles à l'achat sur BenchChem sont spécifiquement conçus pour des études in vitro, qui sont réalisées en dehors des organismes vivants. Les études in vitro, dérivées du terme latin "in verre", impliquent des expériences réalisées dans des environnements de laboratoire contrôlés à l'aide de cellules ou de tissus. Il est important de noter que ces produits ne sont pas classés comme médicaments et n'ont pas reçu l'approbation de la FDA pour la prévention, le traitement ou la guérison de toute condition médicale, affection ou maladie. Nous devons souligner que toute forme d'introduction corporelle de ces produits chez les humains ou les animaux est strictement interdite par la loi. Il est essentiel de respecter ces directives pour assurer la conformité aux normes légales et éthiques en matière de recherche et d'expérimentation.