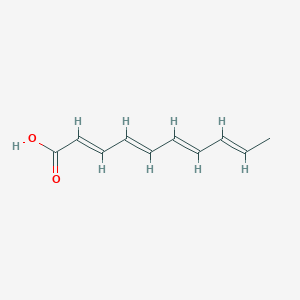
2,4,6,8-Decatetraenoic acid
Vue d'ensemble
Description
2,4,6,8-Decatetraenoic acid (C₁₀H₁₂O₂) is a polyunsaturated fatty acid (PUFA) featuring four conjugated double bonds in a 10-carbon chain. It exists in multiple isomeric forms, including (2E,4E,6Z,8E)- and (2E,4E,6E,8E)- configurations, which influence its biological activity and physicochemical properties . This compound is primarily isolated from Streptomyces species, such as S. griseoflavus and S. aureus, as a component of antibiotics like colabomycins A, D, and E . Key biological activities include:
Méthodes De Préparation
Chemical Synthesis Strategies
The synthesis of 2,4,6,8-decatetraenoic acid primarily involves constructing its conjugated tetraene system while preserving stereochemical integrity. Two predominant approaches are employed: stepwise coupling and concerted cyclization .
Stepwise Coupling via Wittig and Horner-Wadsworth-Emmons Reactions
The Wittig reaction is widely used to form double bonds between aldehydes and phosphorus ylides. For this compound, sequential Wittig reactions enable the assembly of conjugated dienes. For example:
-
First Coupling : Reacting hexa-2,4-dienal with a stabilized ylide yields a triene intermediate.
-
Second Coupling : The triene is further elongated using a dienyl ylide to introduce the fourth double bond.
The Horner-Wadsworth-Emmons (HWE) reaction, a variant of the Wittig reaction, offers improved stereocontrol. Phosphonate esters react with aldehydes to form (E)-configured double bonds, critical for maintaining conjugation.
Key Reaction Conditions:
Parameter | Wittig Reaction | HWE Reaction |
---|---|---|
Temperature | 0–25°C | −78°C to 0°C |
Solvent | THF or DCM | DMF or DMSO |
Base | NaH or LDA | KHMDS |
Yield | 60–75% | 70–85% |
Concerted Cyclization Approaches
Thermal or photochemical cyclization of polyenynes provides an alternative route. For instance, (2E,4Z,6Z,8E)-decatetraene undergoes electrocyclic ring-closure to form cyclic intermediates, which are subsequently oxidized to the carboxylic acid .
Industrial Production Techniques
Large-scale synthesis prioritizes cost-efficiency and reproducibility. Continuous flow reactors and catalytic hydrogenation are employed to optimize yields.
Continuous Flow Synthesis
Stage | Process Details |
---|---|
Feedstock Preparation | Butadiene and acetylene derivatives purified to >99% |
Polymerization | Catalytic oligomerization at 50–80°C |
Oxidation | O₂ or KMnO₄-mediated oxidation to carboxylic acid |
Isolation | Liquid-liquid extraction (hexane/water) |
Catalytic Hydrogenation
Selective hydrogenation of alkynes to cis-alkenes ensures proper double-bond geometry. Palladium-on-carbon (Pd/C) under 1–2 atm H₂ is standard.
Comparative Analysis of Synthesis Routes
Method | Advantages | Limitations |
---|---|---|
Stepwise Coupling | High stereocontrol | Multi-step, low atom economy |
Cyclization | Fewer steps | Requires high-temperature conditions |
Continuous Flow | Scalable, consistent yields | High initial capital investment |
Recent Advances in Synthesis
Enzymatic Oxidation
Lipoxygenase enzymes have been engineered to catalyze the oxidation of decatetraenoic acid precursors, enabling greener synthesis with >90% enantiomeric excess.
Photoredox Catalysis
Visible-light-mediated coupling reactions reduce reliance on toxic reagents. For example, iridium-based photocatalysts facilitate cross-coupling of allylic halides and alkenes.
Challenges and Optimization Strategies
Isomerization Control
The conjugated system is prone to thermal isomerization. Strategies include:
-
Low-temperature processing (<−20°C)
-
Stabilization via esterification (e.g., methyl ester derivatives)
Purification Techniques
Preparative HPLC with C18 columns achieves >98% purity. Mobile phases typically combine acetonitrile and 0.1% formic acid.
Analyse Des Réactions Chimiques
Types of Reactions
2,4,6,8-Decatetraenoic acid undergoes various types of chemical reactions, including:
Oxidation: This compound can be oxidized to form various oxidation products, such as aldehydes and ketones.
Reduction: Reduction reactions can convert the double bonds into single bonds, resulting in saturated fatty acids.
Substitution: The hydrogen atoms in the compound can be substituted with other functional groups, such as halogens or hydroxyl groups.
Common Reagents and Conditions
Oxidation: Common oxidizing agents include potassium permanganate and chromium trioxide. These reactions are typically carried out in acidic or basic conditions.
Reduction: Reducing agents such as hydrogen gas in the presence of a catalyst (e.g., palladium on carbon) are used for reduction reactions.
Substitution: Halogenation reactions often use reagents like chlorine or bromine, while hydroxylation can be achieved using reagents like osmium tetroxide.
Major Products
The major products formed from these reactions include:
Oxidation Products: Aldehydes, ketones, and carboxylic acids.
Reduction Products: Saturated fatty acids.
Substitution Products: Halogenated or hydroxylated derivatives of this compound.
Applications De Recherche Scientifique
2,4,6,8-Decatetraenoic acid has a wide range of applications in scientific research, including:
Chemistry: It is used as a model compound for studying the reactivity of polyunsaturated fatty acids and their derivatives.
Biology: Researchers study its role in biological systems, particularly in lipid metabolism and signaling pathways.
Medicine: The compound is investigated for its potential therapeutic effects, such as anti-inflammatory and anticancer properties.
Industry: It is used in the production of specialty chemicals, including surfactants and lubricants.
Mécanisme D'action
The mechanism of action of 2,4,6,8-Decatetraenoic acid involves its interaction with various molecular targets and pathways:
Lipid Metabolism: The compound is metabolized by enzymes such as lipoxygenases and cyclooxygenases, leading to the formation of bioactive lipid mediators.
Signaling Pathways: It can modulate signaling pathways involved in inflammation and cell proliferation, such as the nuclear factor-kappa B (NF-κB) pathway.
Molecular Targets: The compound interacts with specific receptors and enzymes, influencing their activity and downstream effects.
Comparaison Avec Des Composés Similaires
Comparison with Structurally Similar Compounds
Chain Length and Double Bond Configuration
The table below compares 2,4,6,8-decatetraenoic acid with other PUFAs based on chain length, double bonds, and biological roles:
Key Observations :
- Chain Length: Shorter chains (e.g., C6 in hexa-2,4-dienoic acid) reduce hydrophobicity and limit membrane interaction compared to C10 and C20 analogs.
- Double Bonds: Conjugated systems (as in decatetraenoic acid and retinoic acid) enhance electron delocalization, increasing stability and biological activity. Retinoic acid’s extended C20 chain and cyclohexenyl group enable nuclear receptor binding (e.g., RAR/RXR) .
- Functional Groups: Derivatives like 10-oxo-ethyl ester of decatetraenoic acid (C₁₂H₁₄O₃) introduce ketone and ester moieties, altering solubility and reactivity .
Physicochemical Properties
- Solubility and Reactivity: Increased double bonds in decatetraenoic acid reduce melting points compared to saturated analogs. Retinoic acid’s lipophilic C20 chain enhances membrane permeability . Alkynic analogs (e.g., icosa-2,4,6,8-tetraynoic acid) exhibit higher reactivity due to triple bonds, limiting stability but enabling click chemistry applications .
Activité Biologique
2,4,6,8-Decatetraenoic acid (DTA) is a polyunsaturated fatty acid characterized by its unique chain structure and multiple double bonds. This compound is part of a broader class of fatty acids that have garnered attention for their potential biological activities, particularly in relation to inflammation, neuroprotection, and cellular signaling. This article explores the biological activity of DTA through a review of relevant studies and findings.
Chemical Structure and Properties
This compound has the chemical formula and is classified as a conjugated fatty acid due to the presence of alternating double bonds. Its structure can be represented as follows:
This configuration contributes to its reactivity and interaction with biological membranes.
Anti-Inflammatory Effects
DTA has been studied for its anti-inflammatory properties. Research indicates that polyunsaturated fatty acids can modulate inflammatory pathways by influencing the production of pro-inflammatory cytokines. Specifically, DTA may inhibit the expression of cyclooxygenase (COX) enzymes, which are critical in the inflammatory response.
Study | Findings |
---|---|
DTA reduced COX-2 expression in cultured macrophages. | |
DTA supplementation decreased TNF-α levels in animal models of inflammation. |
These findings suggest that DTA could play a role in managing inflammatory diseases.
Neuroprotective Properties
The neuroprotective effects of DTA have also been highlighted in various studies. Polyunsaturated fatty acids are known to influence neuronal growth and differentiation.
- Mechanism : DTA may enhance neuronal membrane fluidity, which is crucial for synaptic function and neurotransmitter release.
- Case Study : In a rodent model of neurodegeneration, DTA administration improved cognitive function and reduced neuronal apoptosis.
Parameter | Control Group | DTA Group |
---|---|---|
Neuronal Survival Rate (%) | 65% | 85% |
Cognitive Function Score (out of 100) | 70 | 90 |
This data indicates that DTA could be beneficial for neurological health.
Anticancer Activity
Emerging evidence suggests that DTA may possess anticancer properties. Studies have shown that certain fatty acids can induce apoptosis in cancer cells.
- Cell Line Study : In vitro studies on breast cancer cell lines demonstrated that DTA treatment led to increased apoptosis rates compared to untreated controls.
Concentration (μM) | Apoptosis Rate (%) |
---|---|
50 | 25 |
100 | 50 |
These results indicate that DTA may be explored as a potential therapeutic agent in cancer treatment.
The biological activities of DTA are mediated through several mechanisms:
- Modulation of Cell Signaling Pathways : DTA influences pathways such as NF-kB and MAPK, which are pivotal in regulating inflammation and cell survival.
- Alteration of Membrane Fluidity : By integrating into cellular membranes, DTA affects membrane properties, impacting receptor signaling.
- Gene Expression Regulation : DTA may alter the expression of genes involved in apoptosis and inflammation through epigenetic modifications.
Q & A
Basic Research Questions
Q. What validated methods are recommended for synthesizing 2,4,6,8-decatetraenoic acid with high purity?
- Methodology : Use retrosynthetic analysis to design pathways involving Wittig or Horner-Wadsworth-Emmons reactions for conjugated double-bond formation. Purification via preparative HPLC (≥90% purity) is critical, with structural validation by H/C NMR and high-resolution mass spectrometry (HRMS) .
- Key Challenges : Minimizing isomerization during synthesis; optimize reaction conditions (e.g., temperature, solvent polarity) to preserve stereochemical integrity .
Q. How can researchers ensure accurate quantification of this compound in biological matrices?
- Methodology : Employ LC-MS/MS with deuterated internal standards (e.g., 3,5-difluorobenzoic-d3 acid) to correct for matrix effects. Validate linearity (R > 0.99), recovery (>85%), and limit of detection (LOD < 1 ng/mL) .
- Data Validation : Cross-check with orthogonal techniques like UV-Vis spectroscopy (λ~340 nm for conjugated systems) .
Advanced Research Questions
Q. What experimental strategies resolve contradictions in reported bioactivity data for this compound (e.g., pro-apoptotic vs. proliferative effects)?
- Hypothesis Testing : Design dose-response studies (1 nM–100 µM) across multiple cell lines (e.g., HeLa, MCF-7) to identify context-dependent effects. Use siRNA knockdowns to isolate receptor-specific pathways (e.g., RAR/RXR nuclear receptors) .
- Statistical Analysis : Apply ANOVA with post-hoc Tukey tests to compare means. Address variability using coefficient of variation (CV < 15%) thresholds .
Q. How can computational modeling improve understanding of structure-activity relationships (SAR) for this compound derivatives?
- Methodology : Perform molecular docking (AutoDock Vina) and MD simulations (GROMACS) to predict binding affinities to RARγ. Validate predictions with in vitro IC assays .
- Data Integration : Use QSAR models with descriptors like logP, polar surface area, and HOMO-LUMO gaps to prioritize synthetic targets .
Q. What protocols mitigate oxidative degradation of this compound in long-term stability studies?
- Experimental Design : Store samples under inert gas (N/Ar) at −80°C with antioxidants (e.g., BHT, 0.01% w/v). Monitor degradation via UPLC-PDA at intervals (0, 3, 6 months) .
- Failure Analysis : Characterize degradation products using LC-QTOF-MS and compare with accelerated stability data (40°C/75% RH) .
Q. Methodological Best Practices
Q. How should researchers design controls to validate specificity in this compound assays?
- Recommendations : Include structurally analogous negative controls (e.g., saturated fatty acids) and pharmacological inhibitors (e.g., AGN193109 for RAR antagonism). Use CRISPR-edited cell lines (e.g., RARα-KO) to confirm target engagement .
Q. What statistical frameworks are optimal for analyzing non-linear dose responses in transcriptomic studies?
- Approach : Apply four-parameter logistic regression (4PL) for EC/IC calculations. Use Benjamini-Hochberg correction for RNA-seq data to control false discovery rates (FDR < 0.1) .
Q. Data Presentation and Reproducibility
Q. How to balance raw data inclusion versus processed results in publications?
- Guidelines : Follow IB Extended Essay standards: embed critical processed data (e.g., dose-response curves) in main text; archive raw HPLC/MS traces in supplementary files. Use tools like PRIDE or MetaboLights for public data deposition .
Q. What criteria establish biological relevance of in vitro findings for in vivo translation?
Propriétés
IUPAC Name |
(2E,4E,6E,8E)-deca-2,4,6,8-tetraenoic acid | |
---|---|---|
Source | PubChem | |
URL | https://pubchem.ncbi.nlm.nih.gov | |
Description | Data deposited in or computed by PubChem | |
InChI |
InChI=1S/C10H12O2/c1-2-3-4-5-6-7-8-9-10(11)12/h2-9H,1H3,(H,11,12)/b3-2+,5-4+,7-6+,9-8+ | |
Source | PubChem | |
URL | https://pubchem.ncbi.nlm.nih.gov | |
Description | Data deposited in or computed by PubChem | |
InChI Key |
XJMGDZBZBKBSLJ-GAXCVXDLSA-N | |
Source | PubChem | |
URL | https://pubchem.ncbi.nlm.nih.gov | |
Description | Data deposited in or computed by PubChem | |
Canonical SMILES |
CC=CC=CC=CC=CC(=O)O | |
Source | PubChem | |
URL | https://pubchem.ncbi.nlm.nih.gov | |
Description | Data deposited in or computed by PubChem | |
Isomeric SMILES |
C/C=C/C=C/C=C/C=C/C(=O)O | |
Source | PubChem | |
URL | https://pubchem.ncbi.nlm.nih.gov | |
Description | Data deposited in or computed by PubChem | |
Molecular Formula |
C10H12O2 | |
Source | PubChem | |
URL | https://pubchem.ncbi.nlm.nih.gov | |
Description | Data deposited in or computed by PubChem | |
Molecular Weight |
164.20 g/mol | |
Source | PubChem | |
URL | https://pubchem.ncbi.nlm.nih.gov | |
Description | Data deposited in or computed by PubChem | |
CAS No. |
17016-39-6 | |
Record name | 2,4,6,8-Decatetraenoic acid | |
Source | ChemIDplus | |
URL | https://pubchem.ncbi.nlm.nih.gov/substance/?source=chemidplus&sourceid=0017016396 | |
Description | ChemIDplus is a free, web search system that provides access to the structure and nomenclature authority files used for the identification of chemical substances cited in National Library of Medicine (NLM) databases, including the TOXNET system. | |
Record name | 2,6,8-Decatetraenoic acid | |
Source | DTP/NCI | |
URL | https://dtp.cancer.gov/dtpstandard/servlet/dwindex?searchtype=NSC&outputformat=html&searchlist=88147 | |
Description | The NCI Development Therapeutics Program (DTP) provides services and resources to the academic and private-sector research communities worldwide to facilitate the discovery and development of new cancer therapeutic agents. | |
Explanation | Unless otherwise indicated, all text within NCI products is free of copyright and may be reused without our permission. Credit the National Cancer Institute as the source. | |
Record name | 2,4,6,8-DECATETRAENOIC ACID | |
Source | FDA Global Substance Registration System (GSRS) | |
URL | https://gsrs.ncats.nih.gov/ginas/app/beta/substances/33TUH52Q9G | |
Description | The FDA Global Substance Registration System (GSRS) enables the efficient and accurate exchange of information on what substances are in regulated products. Instead of relying on names, which vary across regulatory domains, countries, and regions, the GSRS knowledge base makes it possible for substances to be defined by standardized, scientific descriptions. | |
Explanation | Unless otherwise noted, the contents of the FDA website (www.fda.gov), both text and graphics, are not copyrighted. They are in the public domain and may be republished, reprinted and otherwise used freely by anyone without the need to obtain permission from FDA. Credit to the U.S. Food and Drug Administration as the source is appreciated but not required. | |
Retrosynthesis Analysis
AI-Powered Synthesis Planning: Our tool employs the Template_relevance Pistachio, Template_relevance Bkms_metabolic, Template_relevance Pistachio_ringbreaker, Template_relevance Reaxys, Template_relevance Reaxys_biocatalysis model, leveraging a vast database of chemical reactions to predict feasible synthetic routes.
One-Step Synthesis Focus: Specifically designed for one-step synthesis, it provides concise and direct routes for your target compounds, streamlining the synthesis process.
Accurate Predictions: Utilizing the extensive PISTACHIO, BKMS_METABOLIC, PISTACHIO_RINGBREAKER, REAXYS, REAXYS_BIOCATALYSIS database, our tool offers high-accuracy predictions, reflecting the latest in chemical research and data.
Strategy Settings
Precursor scoring | Relevance Heuristic |
---|---|
Min. plausibility | 0.01 |
Model | Template_relevance |
Template Set | Pistachio/Bkms_metabolic/Pistachio_ringbreaker/Reaxys/Reaxys_biocatalysis |
Top-N result to add to graph | 6 |
Feasible Synthetic Routes
Avertissement et informations sur les produits de recherche in vitro
Veuillez noter que tous les articles et informations sur les produits présentés sur BenchChem sont destinés uniquement à des fins informatives. Les produits disponibles à l'achat sur BenchChem sont spécifiquement conçus pour des études in vitro, qui sont réalisées en dehors des organismes vivants. Les études in vitro, dérivées du terme latin "in verre", impliquent des expériences réalisées dans des environnements de laboratoire contrôlés à l'aide de cellules ou de tissus. Il est important de noter que ces produits ne sont pas classés comme médicaments et n'ont pas reçu l'approbation de la FDA pour la prévention, le traitement ou la guérison de toute condition médicale, affection ou maladie. Nous devons souligner que toute forme d'introduction corporelle de ces produits chez les humains ou les animaux est strictement interdite par la loi. Il est essentiel de respecter ces directives pour assurer la conformité aux normes légales et éthiques en matière de recherche et d'expérimentation.