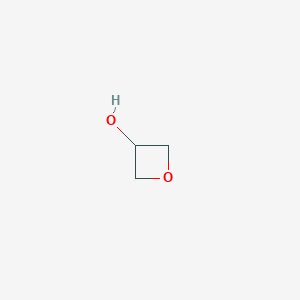
Oxetan-3-ol
Vue d'ensemble
Description
Oxetan-3-ol is a heterocyclic organic compound characterized by a four-membered ring containing three carbon atoms and one oxygen atom. This compound is notable for its unique structural properties, which include significant ring strain due to the small ring size. This compound is often used as a building block in synthetic and medicinal chemistry due to its ability to undergo various chemical transformations.
Applications De Recherche Scientifique
Oxetan-3-ol has a wide range of applications in scientific research:
Mécanisme D'action
Target of Action
Oxetan-3-ol is a versatile compound used in organic synthesis . It serves as an isostere of the carbonyl moiety, suggesting that it may be considered as a potential surrogate of the carboxylic acid functional group . This makes it a valuable tool in the development of new pharmaceuticals .
Mode of Action
The mode of action of this compound involves a highly regioselective ring opening reaction of terminal epoxides . This process is catalyzed by tetrabutylammonium bromide and results in the synthesis of a series of β-hydroxy esters . The formation of the oxetane ring from an epoxide requires a certain amount of activation energy, and therefore, moderate heating is required .
Biochemical Pathways
The biochemical pathways involved in the action of this compound are primarily related to its role in the synthesis of other compounds. For instance, it can be prepared from a β-hydroxy ester intermediate via ring opening in epichlorohydrin . This process is crucial for the synthesis of natural products, pharmaceuticals, and fragrances .
Result of Action
The result of the action of this compound is the efficient production of a range of β-hydroxy esters . These esters are crucial precursors for the synthesis of various compounds, including natural products, pharmaceuticals, and fragrances .
Action Environment
The action of this compound is influenced by various environmental factors. Additionally, the formation of the oxetane ring from an epoxide requires a certain amount of activation energy, indicating that temperature is a key factor in the reaction .
Analyse Biochimique
Biochemical Properties
Oxetan-3-ol is involved in various biochemical reactions. It interacts with a range of enzymes, proteins, and other biomolecules . The nature of these interactions is complex and often involves the formation of high-energy oxygen-containing non-aromatic heterocycles .
Cellular Effects
The effects of this compound on various types of cells and cellular processes are diverse. It influences cell function, including impacts on cell signaling pathways, gene expression, and cellular metabolism .
Molecular Mechanism
This compound exerts its effects at the molecular level through a variety of mechanisms. These include binding interactions with biomolecules, enzyme inhibition or activation, and changes in gene expression .
Temporal Effects in Laboratory Settings
In laboratory settings, the effects of this compound change over time. This includes information on the product’s stability, degradation, and any long-term effects on cellular function observed in in vitro or in vivo studies .
Dosage Effects in Animal Models
The effects of this compound vary with different dosages in animal models . This includes any threshold effects observed in these studies, as well as any toxic or adverse effects at high doses .
Metabolic Pathways
This compound is involved in various metabolic pathways. It interacts with several enzymes or cofactors, and can also affect metabolic flux or metabolite levels .
Transport and Distribution
This compound is transported and distributed within cells and tissues in a complex manner. This includes any transporters or binding proteins that it interacts with, as well as any effects on its localization or accumulation .
Subcellular Localization
The subcellular localization of this compound and its effects on activity or function are diverse. This includes any targeting signals or post-translational modifications that direct it to specific compartments or organelles .
Méthodes De Préparation
Synthetic Routes and Reaction Conditions: Oxetan-3-ol can be synthesized through several methods. One common approach involves the ring-closure reaction of epoxy chloropropane. This method includes the opening of epoxy chloropropane, followed by esterification, electrophilic reaction, and finally, ring-closure to form this compound . Another method involves the gold-catalyzed oxidative cyclization of propargyl alcohol .
Industrial Production Methods: Industrial production of this compound typically involves the use of commercially available starting materials such as epoxy chloropropane. The process is optimized to achieve high yields and purity, making it suitable for large-scale production .
Analyse Des Réactions Chimiques
Types of Reactions: Oxetan-3-ol undergoes various chemical reactions, including:
Oxidation: this compound can be oxidized to form oxetan-3-one, which is a valuable intermediate in synthetic chemistry.
Reduction: Reduction of this compound can yield different derivatives, such as 3-aminooxetane.
Substitution: The hydroxyl group in this compound can be substituted with other functional groups through nucleophilic substitution reactions.
Common Reagents and Conditions:
Oxidation: Common oxidizing agents include peroxy acids and other strong oxidizers.
Reduction: Reducing agents such as lithium aluminum hydride are often used.
Substitution: Nucleophiles like Grignard reagents can be employed for substitution reactions.
Major Products:
Oxetan-3-one: Formed through oxidation.
3-Aminooxetane: Formed through reduction.
Various substituted oxetanes: Formed through nucleophilic substitution.
Comparaison Avec Des Composés Similaires
Oxetan-3-one: A closely related compound used as an intermediate in various synthetic pathways.
3-Oximinooxetane: An important precursor for energetic materials.
Thietan-3-ol: Another four-membered ring compound with sulfur instead of oxygen, used in similar applications.
Uniqueness of Oxetan-3-ol: this compound is unique due to its high ring strain and the presence of a hydroxyl group, which makes it highly reactive and versatile in synthetic chemistry. Its ability to undergo various chemical transformations and its role as a building block in drug discovery highlight its importance in both academic and industrial research .
Propriétés
IUPAC Name |
oxetan-3-ol | |
---|---|---|
Source | PubChem | |
URL | https://pubchem.ncbi.nlm.nih.gov | |
Description | Data deposited in or computed by PubChem | |
InChI |
InChI=1S/C3H6O2/c4-3-1-5-2-3/h3-4H,1-2H2 | |
Source | PubChem | |
URL | https://pubchem.ncbi.nlm.nih.gov | |
Description | Data deposited in or computed by PubChem | |
InChI Key |
QMLWSAXEQSBAAQ-UHFFFAOYSA-N | |
Source | PubChem | |
URL | https://pubchem.ncbi.nlm.nih.gov | |
Description | Data deposited in or computed by PubChem | |
Canonical SMILES |
C1C(CO1)O | |
Source | PubChem | |
URL | https://pubchem.ncbi.nlm.nih.gov | |
Description | Data deposited in or computed by PubChem | |
Molecular Formula |
C3H6O2 | |
Source | PubChem | |
URL | https://pubchem.ncbi.nlm.nih.gov | |
Description | Data deposited in or computed by PubChem | |
DSSTOX Substance ID |
DTXSID30433064 | |
Record name | Oxetan-3-ol | |
Source | EPA DSSTox | |
URL | https://comptox.epa.gov/dashboard/DTXSID30433064 | |
Description | DSSTox provides a high quality public chemistry resource for supporting improved predictive toxicology. | |
Molecular Weight |
74.08 g/mol | |
Source | PubChem | |
URL | https://pubchem.ncbi.nlm.nih.gov | |
Description | Data deposited in or computed by PubChem | |
CAS No. |
7748-36-9 | |
Record name | Oxetan-3-ol | |
Source | EPA DSSTox | |
URL | https://comptox.epa.gov/dashboard/DTXSID30433064 | |
Description | DSSTox provides a high quality public chemistry resource for supporting improved predictive toxicology. | |
Record name | oxetan-3-ol | |
Source | European Chemicals Agency (ECHA) | |
URL | https://echa.europa.eu/information-on-chemicals | |
Description | The European Chemicals Agency (ECHA) is an agency of the European Union which is the driving force among regulatory authorities in implementing the EU's groundbreaking chemicals legislation for the benefit of human health and the environment as well as for innovation and competitiveness. | |
Explanation | Use of the information, documents and data from the ECHA website is subject to the terms and conditions of this Legal Notice, and subject to other binding limitations provided for under applicable law, the information, documents and data made available on the ECHA website may be reproduced, distributed and/or used, totally or in part, for non-commercial purposes provided that ECHA is acknowledged as the source: "Source: European Chemicals Agency, http://echa.europa.eu/". Such acknowledgement must be included in each copy of the material. ECHA permits and encourages organisations and individuals to create links to the ECHA website under the following cumulative conditions: Links can only be made to webpages that provide a link to the Legal Notice page. | |
Retrosynthesis Analysis
AI-Powered Synthesis Planning: Our tool employs the Template_relevance Pistachio, Template_relevance Bkms_metabolic, Template_relevance Pistachio_ringbreaker, Template_relevance Reaxys, Template_relevance Reaxys_biocatalysis model, leveraging a vast database of chemical reactions to predict feasible synthetic routes.
One-Step Synthesis Focus: Specifically designed for one-step synthesis, it provides concise and direct routes for your target compounds, streamlining the synthesis process.
Accurate Predictions: Utilizing the extensive PISTACHIO, BKMS_METABOLIC, PISTACHIO_RINGBREAKER, REAXYS, REAXYS_BIOCATALYSIS database, our tool offers high-accuracy predictions, reflecting the latest in chemical research and data.
Strategy Settings
Precursor scoring | Relevance Heuristic |
---|---|
Min. plausibility | 0.01 |
Model | Template_relevance |
Template Set | Pistachio/Bkms_metabolic/Pistachio_ringbreaker/Reaxys/Reaxys_biocatalysis |
Top-N result to add to graph | 6 |
Feasible Synthetic Routes
Q1: What makes oxetan-3-ol interesting for medicinal chemistry?
A2: this compound has shown promise as a potential bioisostere for the carboxylic acid functional group []. This means it can mimic the properties of a carboxylic acid in a biological context, potentially leading to new drug candidates. This property makes this compound and its derivatives particularly interesting for drug design, as they could offer improved pharmacological profiles compared to their carboxylic acid counterparts.
Q2: Can you provide an example of how this compound has been explored as a carboxylic acid bioisostere?
A3: Researchers have investigated the potential of this compound, thietan-3-ol, and their derivatives as bioisosteres of the carboxylic acid group by synthesizing and evaluating a series of model compounds []. One study focused on developing ibuprofen analogs, where the carboxylic acid moiety of ibuprofen was replaced with these novel structural units. These analogs were then tested for their ability to inhibit eicosanoid biosynthesis in vitro, a key mechanism of action for ibuprofen's anti-inflammatory effects.
Q3: What is the significance of exploring 3-azidooxetane as a potential replacement for glycidyl azide polymer (GAP)?
A4: Glycidyl azide polymer (GAP) is a widely used energetic binder, but its synthesis can lead to polymers with different terminal groups due to the two possible ring-opening modes of the glycidyl azide monomer []. This can result in inconsistent curing properties. 3-Azidooxetane offers a solution as it only forms polymers with primary hydroxyl end groups due to its single ring-opening mode. This controlled polymerization could lead to more homogeneous materials. Furthermore, poly(3-azidooxetane) shares a similar repeating unit with GAP, potentially offering comparable performance characteristics [].
Q4: How are researchers characterizing and evaluating the properties of 3-azidooxetane and its polymer?
A5: Researchers are employing a range of techniques to characterize 3-azidooxetane and poly(3-azidooxetane). These include vibrational and multinuclear NMR spectroscopy (1H, 13C, 14N), differential scanning calorimetry, and elemental analysis []. Additionally, the sensitivity of 3-azidooxetane towards external stimuli like shock and friction is being assessed using standardized BAM procedures []. Theoretical calculations using the EXPLO5 thermochemical code are also employed to predict the energetic performance of these compounds [].
Avertissement et informations sur les produits de recherche in vitro
Veuillez noter que tous les articles et informations sur les produits présentés sur BenchChem sont destinés uniquement à des fins informatives. Les produits disponibles à l'achat sur BenchChem sont spécifiquement conçus pour des études in vitro, qui sont réalisées en dehors des organismes vivants. Les études in vitro, dérivées du terme latin "in verre", impliquent des expériences réalisées dans des environnements de laboratoire contrôlés à l'aide de cellules ou de tissus. Il est important de noter que ces produits ne sont pas classés comme médicaments et n'ont pas reçu l'approbation de la FDA pour la prévention, le traitement ou la guérison de toute condition médicale, affection ou maladie. Nous devons souligner que toute forme d'introduction corporelle de ces produits chez les humains ou les animaux est strictement interdite par la loi. Il est essentiel de respecter ces directives pour assurer la conformité aux normes légales et éthiques en matière de recherche et d'expérimentation.