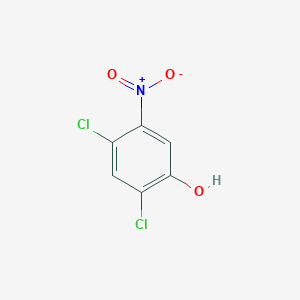
2,4-Dichloro-5-nitrophénol
Vue d'ensemble
Description
2,4-Dichloro-5-nitrophenol is an organic compound with the molecular formula C6H3Cl2NO3. It is a chlorinated nitrophenol derivative, characterized by the presence of two chlorine atoms and one nitro group attached to a phenol ring. This compound is known for its applications in various fields, including chemistry, biology, and industry .
Applications De Recherche Scientifique
2,4-Dichloro-5-nitrophenol has several applications in scientific research:
Mécanisme D'action
Target of Action
2,4-Dichloro-5-nitrophenol (2,4-DCNP) primarily targets certain enzymes in Gram-negative bacteria, such as Cupriavidus sp. CNP-8. The key enzymes involved are HnpAB, a two-component FAD-dependent monooxygenase, and HnpC, a 1,2,4-benzenetriol (BT) 1,2-dioxygenase .
Mode of Action
The compound interacts with its targets by undergoing a series of reactions. HnpAB catalyzes the conversion of 2,4-DCNP to BT via chloro-1,4-benzoquinone . This reaction is crucial for the bacterium to utilize 2,4-DCNP .
Biochemical Pathways
The degradation of 2,4-DCNP occurs via the 1,2,4-benzenetriol (BT) pathway . This pathway is significantly different from the (chloro)hydroquinone pathways reported in other Gram-negative 2,4-DCNP-utilizers . HnpC, a BT 1,2-dioxygenase, catalyzes BT ring-cleavage with the formation of maleylacetate .
Pharmacokinetics
It is known that the compound has high gastrointestinal absorption and can permeate the blood-brain barrier . These properties could potentially impact the bioavailability of the compound.
Result of Action
The result of the compound’s action is the degradation of 2,4-DCNP, a common chlorinated nitrophenol pollutant . This degradation process is crucial for mitigating the environmental impact of such pollutants .
Action Environment
Environmental factors play a significant role in the action of 2,4-DCNP. The compound has been extensively introduced into our surrounding environments mainly by anthropogenic activities . It is resistant to microbial degradation due to the simultaneous existence of electron-withdrawing chlorine and nitro groups on the aromatic ring . Therefore, the efficacy and stability of 2,4-DCNP’s action can be influenced by various environmental conditions.
Analyse Biochimique
Biochemical Properties
2,4-Dichloro-5-nitrophenol plays a role in biochemical reactions, particularly in the inhibition of certain enzymes. It has been shown to interact with enzymes such as phenol sulfotransferase, where it acts as an alternate-substrate inhibitor . This interaction affects the enzyme’s ability to catalyze the transfer of sulfate groups to phenolic compounds, thereby influencing various metabolic pathways.
Cellular Effects
The effects of 2,4-Dichloro-5-nitrophenol on cells include disruption of thyroid hormone synthesis and transport pathways. Studies have shown that exposure to this compound can lead to increased mortality, malformations, and altered thyroid hormone levels in aquatic species . It affects cell signaling pathways and gene expression related to thyroid hormone regulation, impacting cellular metabolism and overall cell function.
Molecular Mechanism
At the molecular level, 2,4-Dichloro-5-nitrophenol exerts its effects through binding interactions with biomolecules and enzyme inhibition. It has been found to disrupt the activity of enzymes involved in thyroid hormone synthesis, such as deiodinases . This disruption leads to altered levels of thyroid hormones, which in turn affect gene expression and cellular processes.
Temporal Effects in Laboratory Settings
In laboratory settings, the effects of 2,4-Dichloro-5-nitrophenol change over time. The compound’s stability and degradation can influence its long-term effects on cellular function. Studies have shown that prolonged exposure to this compound can lead to persistent changes in thyroid hormone levels and gene expression . The stability of 2,4-Dichloro-5-nitrophenol in various environmental conditions also affects its biochemical activity.
Dosage Effects in Animal Models
The effects of 2,4-Dichloro-5-nitrophenol vary with different dosages in animal models. At low doses, the compound may have minimal effects, while higher doses can lead to significant toxicity and adverse effects. For example, exposure to high doses of 2,4-Dichloro-5-nitrophenol has been associated with increased mortality and developmental toxicity in aquatic species . These effects highlight the importance of dosage in determining the compound’s impact on biological systems.
Metabolic Pathways
2,4-Dichloro-5-nitrophenol is involved in metabolic pathways related to the degradation of chlorinated phenols. Bacterial degradation of this compound involves enzymes such as nitroreductases and dioxygenases, which catalyze the breakdown of the nitrophenol structure . These metabolic pathways are crucial for the detoxification and removal of 2,4-Dichloro-5-nitrophenol from the environment.
Transport and Distribution
Within cells and tissues, 2,4-Dichloro-5-nitrophenol is transported and distributed through interactions with transporters and binding proteins. The compound’s distribution affects its localization and accumulation in specific cellular compartments . Understanding these transport mechanisms is essential for predicting the compound’s effects on different tissues and organs.
Subcellular Localization
The subcellular localization of 2,4-Dichloro-5-nitrophenol influences its activity and function. The compound may be directed to specific compartments or organelles through targeting signals or post-translational modifications . These localization patterns determine the sites of action and the biochemical processes affected by 2,4-Dichloro-5-nitrophenol.
Méthodes De Préparation
Synthetic Routes and Reaction Conditions: The synthesis of 2,4-Dichloro-5-nitrophenol typically involves the nitration of 2,4-dichlorophenol. The process includes the following steps:
Nitration Reaction: 2,4-dichlorophenol is treated with a nitrating agent, such as nitric acid, in the presence of a catalyst.
Industrial Production Methods: In industrial settings, the production of 2,4-Dichloro-5-nitrophenol may involve the use of advanced catalysts and optimized reaction conditions to enhance yield and purity. For example, the use of rare earth trifluoromethyl sulfonate catalysts in the presence of co-catalysts and alcoholic solvents has been reported to increase the yield and purity of the product .
Analyse Des Réactions Chimiques
Types of Reactions: 2,4-Dichloro-5-nitrophenol undergoes various chemical reactions, including:
Substitution: The chlorine atoms can be substituted with other functional groups through nucleophilic substitution reactions.
Common Reagents and Conditions:
Substitution: Nucleophiles such as amines or thiols can be used to replace the chlorine atoms under basic conditions.
Major Products:
Comparaison Avec Des Composés Similaires
- 2-Chloro-4-nitrophenol
- 2-Chloro-5-nitrophenol
- 4-Chloro-2-nitrophenol
- 2,6-Dichloro-4-nitrophenol
Comparison: 2,4-Dichloro-5-nitrophenol is unique due to the specific positioning of its chlorine and nitro groups, which influence its reactivity and applications. Compared to other chlorinated nitrophenols, it exhibits distinct chemical properties and biological activities, making it valuable for specific research and industrial purposes .
Activité Biologique
2,4-Dichloro-5-nitrophenol (2,4-DCNP) is a chlorinated nitrophenol compound that has garnered attention due to its significant biological activity and environmental impact. This article explores its biochemical mechanisms, cellular effects, degradation pathways, and relevant case studies that highlight its toxicity and potential for bioremediation.
Target Enzymes
2,4-DCNP primarily targets enzymes in Gram-negative bacteria, notably Cupriavidus sp. CNP-8. Key enzymes involved in its metabolism include:
- HnpAB : A two-component FAD-dependent monooxygenase.
- HnpC : A 1,2,4-benzenetriol dioxygenase.
These enzymes facilitate the conversion of 2,4-DCNP into less harmful metabolites through oxidative and reductive pathways.
2,4-DCNP acts as an inhibitor of various enzymes, including phenol sulfotransferase. Its interaction with these enzymes can disrupt normal biochemical processes, leading to significant physiological effects.
Toxicity and Mortality
Studies have shown that exposure to 2,4-DCNP can lead to increased mortality and developmental malformations in aquatic organisms. In particular, it disrupts thyroid hormone synthesis and transport pathways, affecting growth and development.
Dosage Dependency
The effects of 2,4-DCNP are dosage-dependent. Low doses may exhibit minimal effects, while higher concentrations are associated with severe toxicity. For instance:
- Low Dose : Minimal physiological impact.
- High Dose : Increased mortality rates and developmental issues in aquatic species.
Microbial Metabolism
The degradation of 2,4-DCNP involves specific microbial pathways. Strain CNP-8 has been identified as capable of utilizing 2,4-DCNP as a sole carbon source. The degradation process includes:
- Initial Transformation : Conversion to chlorohydroquinone and hydroquinone intermediates.
- Ring-Cleavage : Facilitated by dioxygenases which cleave the aromatic ring structure .
Kinetics of Degradation
Biodegradation studies indicate that the rate of degradation varies with substrate concentration. For example:
- At concentrations up to 0.6 mM, complete degradation occurs within 90 hours.
- Higher concentrations (>0.7 mM) result in toxic effects that inhibit microbial growth .
Environmental Impact Studies
Research has documented the environmental persistence of 2,4-DCNP and its impact on aquatic ecosystems. A notable study demonstrated that exposure to this compound resulted in altered thyroid hormone levels in fish species, leading to reproductive and developmental challenges .
Bioremediation Potential
Microbial strains capable of degrading 2,4-DCNP have been explored for bioremediation applications. Strain CNP-8 has shown promise in laboratory settings for the simultaneous degradation of multiple nitrophenols under aerobic conditions . This suggests a viable pathway for the remediation of contaminated sites.
Data Tables
Parameter | Value |
---|---|
Molecular Weight | 202.04 g/mol |
Solubility | Soluble in organic solvents |
Toxicity (LC50) | Varies by species; significant at high doses |
Biodegradation Rate | Up to 21.2 μM/h at optimal concentrations |
Propriétés
IUPAC Name |
2,4-dichloro-5-nitrophenol | |
---|---|---|
Source | PubChem | |
URL | https://pubchem.ncbi.nlm.nih.gov | |
Description | Data deposited in or computed by PubChem | |
InChI |
InChI=1S/C6H3Cl2NO3/c7-3-1-4(8)6(10)2-5(3)9(11)12/h1-2,10H | |
Source | PubChem | |
URL | https://pubchem.ncbi.nlm.nih.gov | |
Description | Data deposited in or computed by PubChem | |
InChI Key |
OFAPWTOOMSVMIU-UHFFFAOYSA-N | |
Source | PubChem | |
URL | https://pubchem.ncbi.nlm.nih.gov | |
Description | Data deposited in or computed by PubChem | |
Canonical SMILES |
C1=C(C(=CC(=C1O)Cl)Cl)[N+](=O)[O-] | |
Source | PubChem | |
URL | https://pubchem.ncbi.nlm.nih.gov | |
Description | Data deposited in or computed by PubChem | |
Molecular Formula |
C6H3Cl2NO3 | |
Source | PubChem | |
URL | https://pubchem.ncbi.nlm.nih.gov | |
Description | Data deposited in or computed by PubChem | |
DSSTOX Substance ID |
DTXSID30192624 | |
Record name | Phenol, 2,4-dichloro-5-nitro- | |
Source | EPA DSSTox | |
URL | https://comptox.epa.gov/dashboard/DTXSID30192624 | |
Description | DSSTox provides a high quality public chemistry resource for supporting improved predictive toxicology. | |
Molecular Weight |
208.00 g/mol | |
Source | PubChem | |
URL | https://pubchem.ncbi.nlm.nih.gov | |
Description | Data deposited in or computed by PubChem | |
CAS No. |
39489-77-5 | |
Record name | Phenol, 2,4-dichloro-5-nitro- | |
Source | ChemIDplus | |
URL | https://pubchem.ncbi.nlm.nih.gov/substance/?source=chemidplus&sourceid=0039489775 | |
Description | ChemIDplus is a free, web search system that provides access to the structure and nomenclature authority files used for the identification of chemical substances cited in National Library of Medicine (NLM) databases, including the TOXNET system. | |
Record name | Phenol, 2,4-dichloro-5-nitro- | |
Source | EPA DSSTox | |
URL | https://comptox.epa.gov/dashboard/DTXSID30192624 | |
Description | DSSTox provides a high quality public chemistry resource for supporting improved predictive toxicology. | |
Record name | 2,4-Dichloro-5-nitrophenol | |
Source | European Chemicals Agency (ECHA) | |
URL | https://echa.europa.eu/information-on-chemicals | |
Description | The European Chemicals Agency (ECHA) is an agency of the European Union which is the driving force among regulatory authorities in implementing the EU's groundbreaking chemicals legislation for the benefit of human health and the environment as well as for innovation and competitiveness. | |
Explanation | Use of the information, documents and data from the ECHA website is subject to the terms and conditions of this Legal Notice, and subject to other binding limitations provided for under applicable law, the information, documents and data made available on the ECHA website may be reproduced, distributed and/or used, totally or in part, for non-commercial purposes provided that ECHA is acknowledged as the source: "Source: European Chemicals Agency, http://echa.europa.eu/". Such acknowledgement must be included in each copy of the material. ECHA permits and encourages organisations and individuals to create links to the ECHA website under the following cumulative conditions: Links can only be made to webpages that provide a link to the Legal Notice page. | |
Retrosynthesis Analysis
AI-Powered Synthesis Planning: Our tool employs the Template_relevance Pistachio, Template_relevance Bkms_metabolic, Template_relevance Pistachio_ringbreaker, Template_relevance Reaxys, Template_relevance Reaxys_biocatalysis model, leveraging a vast database of chemical reactions to predict feasible synthetic routes.
One-Step Synthesis Focus: Specifically designed for one-step synthesis, it provides concise and direct routes for your target compounds, streamlining the synthesis process.
Accurate Predictions: Utilizing the extensive PISTACHIO, BKMS_METABOLIC, PISTACHIO_RINGBREAKER, REAXYS, REAXYS_BIOCATALYSIS database, our tool offers high-accuracy predictions, reflecting the latest in chemical research and data.
Strategy Settings
Precursor scoring | Relevance Heuristic |
---|---|
Min. plausibility | 0.01 |
Model | Template_relevance |
Template Set | Pistachio/Bkms_metabolic/Pistachio_ringbreaker/Reaxys/Reaxys_biocatalysis |
Top-N result to add to graph | 6 |
Feasible Synthetic Routes
Q1: Why is there research interest in finding a substitute for tetrachloromethane in the synthesis of 2,4-Dichloro-5-nitrophenol?
A1: Tetrachloromethane, historically used in the synthesis of 2,4-Dichloro-5-nitrophenol, has been identified as an environmental hazard. [] Its use is now prohibited due to its toxicity and ozone-depleting properties. Researchers are exploring alternative solvents like chloroform to mitigate the environmental impact associated with 2,4-Dichloro-5-nitrophenol production. This shift aims to develop a safer and more sustainable synthesis method. []
Q2: What are the potential advantages of using chloroform as a substitute for tetrachloromethane in this synthesis?
A2: While the provided research focuses on the environmental aspect, it hints at potential advantages of using chloroform:
- Yield and Operational Safety: The research investigates the impact of chloroform on the yield of 2,4-Dichloro-5-nitrophenol, implying its viability as a substitute. [] Additionally, the mention of "operation safety" suggests that chloroform might offer a safer alternative in industrial settings. []
- Solvent Recyclability: The research explicitly examines the "recycle of solvent" when using chloroform. [] This suggests the potential for reusing chloroform in the synthesis process, further reducing waste and promoting sustainable practices.
Avertissement et informations sur les produits de recherche in vitro
Veuillez noter que tous les articles et informations sur les produits présentés sur BenchChem sont destinés uniquement à des fins informatives. Les produits disponibles à l'achat sur BenchChem sont spécifiquement conçus pour des études in vitro, qui sont réalisées en dehors des organismes vivants. Les études in vitro, dérivées du terme latin "in verre", impliquent des expériences réalisées dans des environnements de laboratoire contrôlés à l'aide de cellules ou de tissus. Il est important de noter que ces produits ne sont pas classés comme médicaments et n'ont pas reçu l'approbation de la FDA pour la prévention, le traitement ou la guérison de toute condition médicale, affection ou maladie. Nous devons souligner que toute forme d'introduction corporelle de ces produits chez les humains ou les animaux est strictement interdite par la loi. Il est essentiel de respecter ces directives pour assurer la conformité aux normes légales et éthiques en matière de recherche et d'expérimentation.