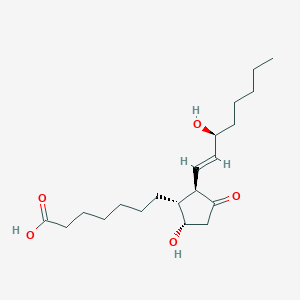
Prostaglandin D1
Vue d'ensemble
Description
Prostaglandin D1 (PGD1) is a member of the prostaglandin family, lipid mediators derived from arachidonic acid or dihomo-γ-linolenic acid (DGLA). PGD1 exhibits dual vasoactive properties, causing contraction and dilation of isolated human arteries, and inhibits ADP-induced platelet aggregation with an IC50 of 320 ng/mL .
Méthodes De Préparation
Corey-Nicolaou Lactonization: A Foundational Approach
The Corey-Nicolaou procedure, a cornerstone in prostaglandin synthesis, has been adapted for PGD1 preparation. This method utilizes δ-lactone and γ-lactone intermediates to construct the cyclopentane core with precise stereocontrol .
Stepwise Lactonization and Functionalization
The synthesis begins with the formation of a mixed anhydride from the C1 carboxylic acid and a protected hydroxyl group (e.g., at C9 or C15). For PGD1, the C11 ketone is introduced early to avoid side reactions. Key steps include:
-
Activation of C1 Acid : Reaction with 2,4,6-trichlorobenzoyl chloride forms a reactive mixed anhydride, enabling nucleophilic attack by the C9 hydroxyl group .
-
Macrolactonization : Intramolecular esterification under dilute conditions yields the 1,9-lactone intermediate. Yields range from 61.6% to 82.4%, depending on protecting groups (e.g., tetrahydropyranyl or silyl ethers) .
-
Oxidation at C11 : Jones oxidation (CrO₃ in H₂SO₄) converts the C11 hydroxyl to a ketone, critical for PGD1’s structure .
Example Protocol :
This method achieves an overall yield of 58–65% but requires meticulous protection-deprotection steps to prevent isomerization .
Chemoenzymatic Synthesis: Bridging Efficiency and Scalability
Recent advances employ chemoenzymatic strategies to streamline PGD1 synthesis. A 2024 study demonstrated a 5-step route starting from bromohydrin, a radical equivalent of Corey lactone .
Enzymatic Resolution and Nickel Catalysis
-
Bromohydrin Formation : Epoxidation of a cyclopentene derivative followed by enzymatic hydrolysis (lipase B) yields enantiopure bromohydrin (98% ee) .
-
Cross-Coupling : Nickel-catalyzed Suzuki-Miyaura coupling installs the ω-side chain (C13–C20) with high regioselectivity.
-
Wittig Reaction : The α-side chain (C1–C8) is appended using a stabilized ylide, completing the carbon skeleton .
Advantages :
-
Reduced reliance on toxic reagents (e.g., chromium oxidants).
Organocatalytic Asymmetric Synthesis
Organocatalysis offers a metal-free alternative for constructing PGD1’s stereocenters. A proline-catalyzed aldol dimerization of succinaldehyde generates the cyclopentane core with >95% enantiomeric excess . Subsequent functionalization introduces the C9 and C15 hydroxyl groups via Sharpless asymmetric dihydroxylation .
Key Reaction :
This method avoids traditional lactonization bottlenecks but requires additional steps to install the ketone and side chains .
Comparative Analysis of Synthetic Methods
Method | Steps | Overall Yield | Key Advantages | Limitations |
---|---|---|---|---|
Corey-Nicolaou | 7–9 | 58–65% | High stereocontrol | Tedious protection steps |
Chemoenzymatic | 5 | 72% | Scalable, eco-friendly | Requires specialized enzymes |
Organocatalytic | 6–7 | 68% | Metal-free, high ee | Limited substrate scope |
Challenges in PGD1 Synthesis
-
Isomerization at C13 : The trans double bond is prone to cis isomerization under acidic or basic conditions, necessitating low-temperature protocols .
-
Ketone Stability : The C11 ketone can undergo undesired reductions; careful choice of oxidizing agents (e.g., Dess-Martin periodinane) mitigates this .
-
Purification : PGD1’s polarity complicates chromatography. Crystallization from hexane/ethyl acetate mixtures improves purity (>98%) .
Analyse Des Réactions Chimiques
Types de réactions : La prostaglandine D1 subit diverses réactions chimiques, notamment l'oxydation, la réduction et la substitution.
Réactifs et conditions courants :
Oxydation : Implique généralement l'utilisation d'agents oxydants tels que le peroxyde d'hydrogène ou l'oxygène moléculaire en présence de catalyseurs.
Réduction : Souvent réalisée à l'aide d'agents réducteurs comme le borohydrure de sodium ou l'hydrure de lithium et d'aluminium.
Substitution : Peut impliquer des réactions d'halogénation utilisant des réactifs comme la N-bromosuccinimide (NBS) en conditions aqueuses.
Principaux produits : Les principaux produits formés à partir de ces réactions comprennent divers dérivés et analogues de prostaglandines, qui sont souvent utilisés en chimie médicinale pour le développement de médicaments .
4. Applications de la recherche scientifique
La prostaglandine D1 a une large gamme d'applications de recherche scientifique :
Chimie : Utilisée comme composé modèle pour étudier les réactions d'oxydation et de cyclisation lipidiques.
5. Mécanisme d'action
La prostaglandine D1 exerce ses effets en se liant à des récepteurs couplés aux protéines G (RCPG) spécifiques. Ces récepteurs activent diverses voies de signalisation intracellulaires, notamment la voie adénylcyclase-AMPc et la voie de la phosphoinositide 3-kinase . L'activation de ces voies conduit à diverses réponses biologiques, telles que la vasodilatation, l'inhibition de l'agrégation plaquettaire et la modulation des processus inflammatoires .
Composés similaires :
- Prostaglandine D2
- Prostaglandine E1
- Prostaglandine F2α
- Prostacycline (PGI2)
- Thromboxane A2
Comparaison : La prostaglandine D1 est unique dans ses interactions spécifiques avec les récepteurs et les effets biologiques qui en résultent. Bien que d'autres prostaglandines, comme la prostaglandine D2 et la prostaglandine E1, jouent également un rôle dans l'inflammation et la régulation vasculaire, la prostaglandine D1 a des affinités de récepteurs et des voies de signalisation distinctes . Cette unicité en fait un composé précieux pour les applications thérapeutiques ciblées et la recherche.
Applications De Recherche Scientifique
Physiological Functions
Vascular Effects
- PGD1 has been shown to elicit both contractile and relaxant responses in isolated human pial arteries, indicating its role in vascular tone regulation. The compound exhibits a small potency but is significant in modulating blood flow and pressure .
Neuroprotective Roles
- Recent studies have highlighted PGD1's potential neuroprotective effects, particularly in the context of stroke. It is involved in the modulation of inflammatory responses and may aid in cognitive recovery post-stroke. Serum levels of resolvin D1, which is related to PGD1, have been associated with improved neurofunctional outcomes following acute ischemic stroke .
Clinical Applications
Cancer Research
- PGD1 has been implicated in cancer biology, particularly through its interactions with cyclin D1, a key regulator of cell cycle progression. Research indicates that PGD1 may influence tumor growth by modulating cyclin D1 expression, thereby affecting cell proliferation and survival .
Therapeutic Potential
Anti-Inflammatory Effects
- As a prostanoid, PGD1 exhibits anti-inflammatory properties that could be harnessed in treating inflammatory diseases. Its ability to regulate immune responses makes it a candidate for therapeutic interventions in conditions such as arthritis or inflammatory bowel disease.
Cardiovascular Health
- Given its role in vascular function, PGD1 may have applications in cardiovascular health management. Its effects on blood vessel relaxation could be beneficial for conditions characterized by impaired vasodilation.
Data Tables
Case Studies
Case Study 1: Stroke Recovery
A recent study found that higher serum levels of resolvin D1 (related to PGD1) were predictive of better neurofunctional recovery in patients post-stroke. This suggests that targeting the pathways involving PGD1 could enhance recovery outcomes in stroke patients .
Case Study 2: Cancer Cell Lines
In vitro studies demonstrated that treatment with PGD1 resulted in decreased cyclin D1 expression in various cancer cell lines. This down-regulation was linked to growth arrest, indicating potential therapeutic strategies for cancer treatment through modulation of prostaglandin signaling pathways .
Mécanisme D'action
Prostaglandin D1 exerts its effects through binding to specific G-protein-coupled receptors (GPCRs). These receptors activate various intracellular signaling pathways, including the adenyl cyclase-cAMP pathway and the phosphoinositide 3-kinase pathway . The activation of these pathways leads to diverse biological responses, such as vasodilation, inhibition of platelet aggregation, and modulation of inflammatory processes .
Comparaison Avec Des Composés Similaires
Structural and Molecular Comparison
Compound | Molecular Formula | Molecular Weight (g/mol) | Key Structural Features |
---|---|---|---|
PGD1 | C20H34O5 | 354.5 | 15-hydroxy group; unsaturated carbon chain |
PGD2 | C20H32O5 | 352.5 | Additional double bond at C13-C14 |
PGE1 | C20H34O5 | 354.5 | 9-keto, 11α-hydroxy groups |
PGF2α | C20H34O5 | 354.5 | 9α,11α-dihydroxy groups |
Key Differences :
- PGD1 and PGD2 differ in double bond positioning, impacting receptor affinity and stability .
- PGE1 and PGF2α exhibit distinct functional groups (keto vs. dihydroxy), leading to divergent biological roles .
Pharmacological Profiles
Critical Notes:
- PGD1’s instability in solvent form necessitates stringent storage conditions .
- PGD2 and PGE1 have more robust clinical applications due to extensive research .
Receptor Targets :
Activité Biologique
Prostaglandin D1 (PGD1) is a member of the prostaglandin family, which are lipid compounds derived from arachidonic acid. PGD1 plays a significant role in various physiological and pathological processes, including inflammation, vascular regulation, and cell proliferation. This article explores the biological activity of PGD1, focusing on its mechanisms of action, receptor interactions, and implications in health and disease.
PGD1 exerts its biological effects primarily through its interaction with specific receptors. The two main receptors involved are:
- DP1 Receptor : The activation of DP1 receptors by PGD1 leads to vasodilation and increased blood flow. This receptor is also implicated in anti-inflammatory responses and modulation of immune cell function .
- DP2 Receptor : While less understood, DP2 receptor activation has been linked to allergic responses and asthma pathophysiology.
Biological Effects
The biological activities of PGD1 can be summarized as follows:
- Vasodilation : PGD1 induces relaxation of vascular smooth muscle, contributing to increased blood flow and reduced blood pressure.
- Anti-inflammatory Effects : PGD1 modulates the immune response by inhibiting pro-inflammatory cytokines and promoting the production of anti-inflammatory mediators.
- Cell Proliferation : Studies indicate that PGD1 may influence cell cycle progression and apoptosis in various cell types, including cancer cells .
Research Findings
Recent studies have elucidated the complex roles of PGD1 in different biological contexts:
Table 1: Summary of Key Research Findings on PGD1
Case Study 1: Cardiovascular Health
A study examined the effects of PGD1 on patients with hypertension. The results indicated that administration of PGD1 analogs led to significant reductions in blood pressure and improved endothelial function. This suggests a potential role for PGD1 in managing hypertension and related cardiovascular conditions.
Case Study 2: Inflammatory Diseases
In a clinical trial involving patients with asthma, treatment with a DP1 agonist showed a reduction in airway inflammation and improved lung function. These findings support the therapeutic potential of targeting PGD1 pathways in respiratory diseases.
Q & A
Basic Research Questions
Q. What are the standard in vitro assays for evaluating PGD1's effects on platelet aggregation, and how are they optimized?
- Methodology : Use ADP-induced platelet aggregation assays with washed human platelets. Optimize agonist concentrations (e.g., 1–5 μM ADP) and pre-incubate PGD1 for 5–10 minutes before adding ADP. Quantify inhibition using IC50 values (e.g., 320 ng/mL for PGD1) via turbidimetric or impedance-based aggregometry .
- Key Considerations : Include controls for solvent effects (e.g., ethanol/DMSO) and validate platelet viability using ATP release assays.
Q. How can researchers quantify PGD1 in complex biological matrices like plasma or tissue homogenates?
- Methodology : Employ liquid chromatography-tandem mass spectrometry (LC-MS/MS) with deuterated internal standards (e.g., d4-PGD1). Optimize sample preparation using solid-phase extraction (SPE) to minimize matrix interference and improve sensitivity .
- Validation : Report limits of detection (LOD), recovery rates (>80%), and inter-day precision (<15% CV).
Q. What experimental models are appropriate for studying PGD1's dual vasoactive effects (contraction/relaxation) in vascular tissues?
- Methodology : Use isolated human arterial rings in organ baths. Pre-contract tissues with norepinephrine (1 μM), then apply PGD1 to assess relaxation. For contraction studies, apply PGD1 to resting vessels. Normalize responses to baseline tension and compare to reference vasodilators (e.g., acetylcholine) .
- Data Interpretation : Analyze dose-response curves to calculate EC50 values and receptor specificity (e.g., DP1 vs. TP receptors).
Q. How can experimental reproducibility be ensured in PGD1 studies?
- Guidelines : Adhere to NIH reporting standards for preclinical research, including detailed protocols for platelet isolation, agonist preparation, and statistical thresholds (e.g., p < 0.05). Provide raw data in supplementary materials and specify software versions for analysis .
Advanced Research Questions
Q. How should researchers address discrepancies in reported IC50 values for PGD1's antiplatelet effects across studies?
- Analysis Framework : Compare variables such as platelet source (human vs. animal), ADP concentrations, and assay conditions (e.g., stirring speed in aggregometry). Use meta-analysis tools to assess heterogeneity and publish negative results to reduce publication bias .
Q. What experimental strategies resolve contradictions in PGD1's vasodilatory vs. vasoconstrictive effects?
- Approach : Investigate receptor subtype interactions using selective antagonists (e.g., BW-A868C for DP1) or CRISPR-edited vascular cells lacking specific receptors. Perform RNA-seq to identify downstream signaling pathways (e.g., cAMP/PKA vs. Rho-kinase) .
Q. How does PGD1 interact with other eicosanoids (e.g., thromboxane A2) in modulating vascular tone or thrombosis?
- Methodology : Co-administer PGD1 with thromboxane A2 mimetics (e.g., U46619) in platelet-rich plasma or arterial rings. Measure cross-talk via calcium flux assays or prostaglandin synthase activity profiling .
Q. What advanced techniques characterize PGD1's metabolic fate in vivo?
- Protocol : Administer isotopically labeled PGD1 (e.g., ¹³C-PGD1) to animal models and analyze metabolites via high-resolution MS. Identify major pathways (e.g., 15-keto-PGD1 formation) and compare to in vitro liver microsome assays .
Q. Which statistical approaches are optimal for analyzing time-dependent PGD1 effects in longitudinal studies?
- Recommendations : Use mixed-effects models to account for intra-subject variability. For small sample sizes, apply non-parametric tests (e.g., Friedman test) and report effect sizes with 95% confidence intervals .
Q. How can researchers develop hypotheses about PGD1's role in disease using frameworks like PICO or FINER?
Propriétés
IUPAC Name |
7-[(1R,2R,5S)-5-hydroxy-2-[(E,3S)-3-hydroxyoct-1-enyl]-3-oxocyclopentyl]heptanoic acid | |
---|---|---|
Source | PubChem | |
URL | https://pubchem.ncbi.nlm.nih.gov | |
Description | Data deposited in or computed by PubChem | |
InChI |
InChI=1S/C20H34O5/c1-2-3-6-9-15(21)12-13-17-16(18(22)14-19(17)23)10-7-4-5-8-11-20(24)25/h12-13,15-18,21-22H,2-11,14H2,1H3,(H,24,25)/b13-12+/t15-,16+,17+,18-/m0/s1 | |
Source | PubChem | |
URL | https://pubchem.ncbi.nlm.nih.gov | |
Description | Data deposited in or computed by PubChem | |
InChI Key |
CIMMACURCPXICP-PNQRDDRVSA-N | |
Source | PubChem | |
URL | https://pubchem.ncbi.nlm.nih.gov | |
Description | Data deposited in or computed by PubChem | |
Canonical SMILES |
CCCCCC(C=CC1C(C(CC1=O)O)CCCCCCC(=O)O)O | |
Source | PubChem | |
URL | https://pubchem.ncbi.nlm.nih.gov | |
Description | Data deposited in or computed by PubChem | |
Isomeric SMILES |
CCCCC[C@@H](/C=C/[C@@H]1[C@H]([C@H](CC1=O)O)CCCCCCC(=O)O)O | |
Source | PubChem | |
URL | https://pubchem.ncbi.nlm.nih.gov | |
Description | Data deposited in or computed by PubChem | |
Molecular Formula |
C20H34O5 | |
Source | PubChem | |
URL | https://pubchem.ncbi.nlm.nih.gov | |
Description | Data deposited in or computed by PubChem | |
DSSTOX Substance ID |
DTXSID80864809 | |
Record name | Prostaglandin D1 | |
Source | EPA DSSTox | |
URL | https://comptox.epa.gov/dashboard/DTXSID80864809 | |
Description | DSSTox provides a high quality public chemistry resource for supporting improved predictive toxicology. | |
Molecular Weight |
354.5 g/mol | |
Source | PubChem | |
URL | https://pubchem.ncbi.nlm.nih.gov | |
Description | Data deposited in or computed by PubChem | |
Physical Description |
Solid | |
Record name | Prostaglandin D1 | |
Source | Human Metabolome Database (HMDB) | |
URL | http://www.hmdb.ca/metabolites/HMDB0005102 | |
Description | The Human Metabolome Database (HMDB) is a freely available electronic database containing detailed information about small molecule metabolites found in the human body. | |
Explanation | HMDB is offered to the public as a freely available resource. Use and re-distribution of the data, in whole or in part, for commercial purposes requires explicit permission of the authors and explicit acknowledgment of the source material (HMDB) and the original publication (see the HMDB citing page). We ask that users who download significant portions of the database cite the HMDB paper in any resulting publications. | |
CAS No. |
17968-82-0 | |
Record name | PGD1 | |
Source | CAS Common Chemistry | |
URL | https://commonchemistry.cas.org/detail?cas_rn=17968-82-0 | |
Description | CAS Common Chemistry is an open community resource for accessing chemical information. Nearly 500,000 chemical substances from CAS REGISTRY cover areas of community interest, including common and frequently regulated chemicals, and those relevant to high school and undergraduate chemistry classes. This chemical information, curated by our expert scientists, is provided in alignment with our mission as a division of the American Chemical Society. | |
Explanation | The data from CAS Common Chemistry is provided under a CC-BY-NC 4.0 license, unless otherwise stated. | |
Record name | Prostaglandin D1 | |
Source | ChemIDplus | |
URL | https://pubchem.ncbi.nlm.nih.gov/substance/?source=chemidplus&sourceid=0017968820 | |
Description | ChemIDplus is a free, web search system that provides access to the structure and nomenclature authority files used for the identification of chemical substances cited in National Library of Medicine (NLM) databases, including the TOXNET system. | |
Record name | Prostaglandin D1 | |
Source | EPA DSSTox | |
URL | https://comptox.epa.gov/dashboard/DTXSID80864809 | |
Description | DSSTox provides a high quality public chemistry resource for supporting improved predictive toxicology. | |
Record name | PROSTAGLANDIN D1 | |
Source | FDA Global Substance Registration System (GSRS) | |
URL | https://gsrs.ncats.nih.gov/ginas/app/beta/substances/FOT50Z88GW | |
Description | The FDA Global Substance Registration System (GSRS) enables the efficient and accurate exchange of information on what substances are in regulated products. Instead of relying on names, which vary across regulatory domains, countries, and regions, the GSRS knowledge base makes it possible for substances to be defined by standardized, scientific descriptions. | |
Explanation | Unless otherwise noted, the contents of the FDA website (www.fda.gov), both text and graphics, are not copyrighted. They are in the public domain and may be republished, reprinted and otherwise used freely by anyone without the need to obtain permission from FDA. Credit to the U.S. Food and Drug Administration as the source is appreciated but not required. | |
Record name | Prostaglandin D1 | |
Source | Human Metabolome Database (HMDB) | |
URL | http://www.hmdb.ca/metabolites/HMDB0005102 | |
Description | The Human Metabolome Database (HMDB) is a freely available electronic database containing detailed information about small molecule metabolites found in the human body. | |
Explanation | HMDB is offered to the public as a freely available resource. Use and re-distribution of the data, in whole or in part, for commercial purposes requires explicit permission of the authors and explicit acknowledgment of the source material (HMDB) and the original publication (see the HMDB citing page). We ask that users who download significant portions of the database cite the HMDB paper in any resulting publications. | |
Retrosynthesis Analysis
AI-Powered Synthesis Planning: Our tool employs the Template_relevance Pistachio, Template_relevance Bkms_metabolic, Template_relevance Pistachio_ringbreaker, Template_relevance Reaxys, Template_relevance Reaxys_biocatalysis model, leveraging a vast database of chemical reactions to predict feasible synthetic routes.
One-Step Synthesis Focus: Specifically designed for one-step synthesis, it provides concise and direct routes for your target compounds, streamlining the synthesis process.
Accurate Predictions: Utilizing the extensive PISTACHIO, BKMS_METABOLIC, PISTACHIO_RINGBREAKER, REAXYS, REAXYS_BIOCATALYSIS database, our tool offers high-accuracy predictions, reflecting the latest in chemical research and data.
Strategy Settings
Precursor scoring | Relevance Heuristic |
---|---|
Min. plausibility | 0.01 |
Model | Template_relevance |
Template Set | Pistachio/Bkms_metabolic/Pistachio_ringbreaker/Reaxys/Reaxys_biocatalysis |
Top-N result to add to graph | 6 |
Feasible Synthetic Routes
Avertissement et informations sur les produits de recherche in vitro
Veuillez noter que tous les articles et informations sur les produits présentés sur BenchChem sont destinés uniquement à des fins informatives. Les produits disponibles à l'achat sur BenchChem sont spécifiquement conçus pour des études in vitro, qui sont réalisées en dehors des organismes vivants. Les études in vitro, dérivées du terme latin "in verre", impliquent des expériences réalisées dans des environnements de laboratoire contrôlés à l'aide de cellules ou de tissus. Il est important de noter que ces produits ne sont pas classés comme médicaments et n'ont pas reçu l'approbation de la FDA pour la prévention, le traitement ou la guérison de toute condition médicale, affection ou maladie. Nous devons souligner que toute forme d'introduction corporelle de ces produits chez les humains ou les animaux est strictement interdite par la loi. Il est essentiel de respecter ces directives pour assurer la conformité aux normes légales et éthiques en matière de recherche et d'expérimentation.