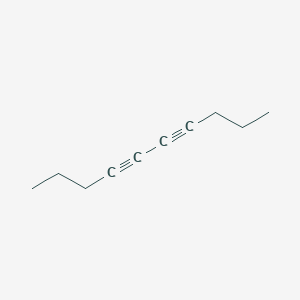
4,6-Décadiyne
Vue d'ensemble
Description
4,6-Decadiyne is an organic compound with the molecular formula C10H14. It is a diacetylene, meaning it contains two triple bonds within its carbon chain. This compound is known for its unique structure and reactivity, making it a subject of interest in various fields of chemistry and materials science.
Applications De Recherche Scientifique
4,6-Decadiyne has several applications in scientific research:
Chemistry: It is used as a building block in the synthesis of more complex organic molecules. Its unique structure makes it valuable in studying reaction mechanisms and developing new synthetic methodologies.
Materials Science: 4,6-Decadiyne is used in the preparation of thin films and polymers. For example, poly 4,6-Decadiyne (PDA) is used in the fabrication of thin films with specific optical and electronic properties.
Biology and Medicine: While specific biological applications are less documented, compounds with similar structures have been studied for their potential biological activities, including antimicrobial and anticancer properties.
Industry: In industrial applications, 4,6-Decadiyne can be used as a precursor for the synthesis of specialty chemicals and materials.
Mécanisme D'action
Target of Action
This compound is a diacetylene, a class of compounds known for their potential applications in various fields due to their unique chemical properties . .
Action Environment
Environmental factors can significantly influence a compound’s action, efficacy, and stabilityIt’s worth noting that handling of 4,6-decadiyne should be done in a well-ventilated place, and contact with skin and eyes should be avoided .
Méthodes De Préparation
Synthetic Routes and Reaction Conditions
4,6-Decadiyne can be synthesized through several methods. One common approach involves the coupling of terminal alkynes. For example, the reaction between 1-bromo-4-pentyne and 1-bromo-5-pentyne in the presence of a palladium catalyst can yield 4,6-Decadiyne. The reaction typically requires a base such as triethylamine and is carried out under an inert atmosphere to prevent oxidation.
Industrial Production Methods
While specific industrial production methods for 4,6-Decadiyne are not widely documented, the general principles of alkyne coupling and the use of palladium catalysts are likely employed. Industrial synthesis would focus on optimizing yield and purity while minimizing costs and environmental impact.
Analyse Des Réactions Chimiques
Types of Reactions
4,6-Decadiyne undergoes various chemical reactions, including:
Oxidation: This compound can be oxidized to form diketones or carboxylic acids, depending on the conditions and reagents used.
Reduction: Hydrogenation of 4,6-Decadiyne can yield alkenes or alkanes, depending on the extent of hydrogenation.
Substitution: The triple bonds in 4,6-Decadiyne can participate in substitution reactions, such as halogenation or hydrohalogenation.
Common Reagents and Conditions
Oxidation: Reagents such as potassium permanganate or ozone can be used for oxidation reactions.
Reduction: Catalysts like palladium on carbon or nickel are commonly used for hydrogenation.
Substitution: Halogenation can be achieved using reagents like bromine or chlorine in the presence of a catalyst.
Major Products
Oxidation: Products can include diketones or carboxylic acids.
Reduction: Products can range from partially hydrogenated alkenes to fully hydrogenated alkanes.
Substitution: Halogenated derivatives of 4,6-Decadiyne are common products.
Comparaison Avec Des Composés Similaires
Similar Compounds
1,3-Butadiyne: Another diacetylene with two triple bonds, but with a shorter carbon chain.
Hexa-1,5-diyne: A diacetylene with a six-carbon chain and two triple bonds.
Octa-1,7-diyne: A diacetylene with an eight-carbon chain and two triple bonds.
Uniqueness
4,6-Decadiyne is unique due to its specific carbon chain length and the position of its triple bonds. This structure imparts distinct reactivity and properties compared to other diacetylenes. Its longer carbon chain provides more flexibility and potential for functionalization, making it a versatile compound in synthetic chemistry and materials science.
Activité Biologique
4,6-Decadiyne is a linear alkyne compound with the molecular formula C₁₀H₁₄. It has garnered attention in the field of biological research due to its diverse pharmacological properties, particularly as an antimicrobial and anticancer agent. This article explores the biological activities associated with 4,6-decadiyne, focusing on its mechanisms of action, relevant case studies, and potential applications in medicine.
Chemical Structure and Properties
4,6-Decadiyne features a unique structure characterized by two triple bonds located at the 4th and 6th carbon positions. This configuration contributes to its reactivity and biological activity. The compound can be represented as follows:
Antimicrobial Properties
Research indicates that 4,6-decadiyne exhibits significant antimicrobial activity against various pathogens. In particular, studies have highlighted its effectiveness against fungal strains isolated from mushrooms. For example:
- Fungal Activity : 4,6-decadiyne has shown antifungal properties against Lentinula edodes, commonly known as shiitake mushroom, which is noted for its medicinal benefits .
- Bacterial Activity : The compound has demonstrated antibacterial effects against Escherichia coli and Staphylococcus aureus, suggesting its potential as a natural preservative or therapeutic agent in treating infections .
Anticancer Effects
The anticancer potential of 4,6-decadiyne is supported by several studies that indicate its ability to inhibit tumor cell proliferation. Notably:
- Cell Line Studies : In vitro studies using human cancer cell lines have shown that 4,6-decadiyne induces apoptosis (programmed cell death) in cancer cells. This effect is mediated through the generation of reactive oxygen species (ROS), leading to oxidative stress within the cells .
- Mechanism of Action : The compound's mechanism involves the disruption of mitochondrial function and activation of caspases, which are crucial for the apoptotic pathway .
Study 1: Antifungal Activity
A study conducted on various alkynyl compounds isolated from mushrooms revealed that 4,6-decadiyne exhibited notable antifungal activity against Lentinula edodes. The Minimum Inhibitory Concentration (MIC) was determined to be low, indicating high potency .
Compound | Source | MIC (µg/mL) |
---|---|---|
4,6-Decadiyne | Lentinula edodes | 15 |
Control (Fluconazole) | - | 10 |
Study 2: Anticancer Activity
Another significant study focused on the cytotoxic effects of 4,6-decadiyne on human breast cancer cell lines (MCF-7). The results showed a dose-dependent decrease in cell viability.
Concentration (µM) | % Cell Viability |
---|---|
0 | 100 |
10 | 75 |
50 | 50 |
100 | 25 |
Propriétés
IUPAC Name |
deca-4,6-diyne | |
---|---|---|
Source | PubChem | |
URL | https://pubchem.ncbi.nlm.nih.gov | |
Description | Data deposited in or computed by PubChem | |
InChI |
InChI=1S/C10H14/c1-3-5-7-9-10-8-6-4-2/h3-6H2,1-2H3 | |
Source | PubChem | |
URL | https://pubchem.ncbi.nlm.nih.gov | |
Description | Data deposited in or computed by PubChem | |
InChI Key |
LIWZSNTUMSGWTF-UHFFFAOYSA-N | |
Source | PubChem | |
URL | https://pubchem.ncbi.nlm.nih.gov | |
Description | Data deposited in or computed by PubChem | |
Canonical SMILES |
CCCC#CC#CCCC | |
Source | PubChem | |
URL | https://pubchem.ncbi.nlm.nih.gov | |
Description | Data deposited in or computed by PubChem | |
Molecular Formula |
C10H14 | |
Source | PubChem | |
URL | https://pubchem.ncbi.nlm.nih.gov | |
Description | Data deposited in or computed by PubChem | |
DSSTOX Substance ID |
DTXSID50167672 | |
Record name | 4,6-Decadiyne | |
Source | EPA DSSTox | |
URL | https://comptox.epa.gov/dashboard/DTXSID50167672 | |
Description | DSSTox provides a high quality public chemistry resource for supporting improved predictive toxicology. | |
Molecular Weight |
134.22 g/mol | |
Source | PubChem | |
URL | https://pubchem.ncbi.nlm.nih.gov | |
Description | Data deposited in or computed by PubChem | |
CAS No. |
16387-71-6 | |
Record name | 4,6-Decadiyne | |
Source | ChemIDplus | |
URL | https://pubchem.ncbi.nlm.nih.gov/substance/?source=chemidplus&sourceid=0016387716 | |
Description | ChemIDplus is a free, web search system that provides access to the structure and nomenclature authority files used for the identification of chemical substances cited in National Library of Medicine (NLM) databases, including the TOXNET system. | |
Record name | 4,6-Decadiyne | |
Source | EPA DSSTox | |
URL | https://comptox.epa.gov/dashboard/DTXSID50167672 | |
Description | DSSTox provides a high quality public chemistry resource for supporting improved predictive toxicology. | |
Record name | 4,6-Decadiyne | |
Source | European Chemicals Agency (ECHA) | |
URL | https://echa.europa.eu/information-on-chemicals | |
Description | The European Chemicals Agency (ECHA) is an agency of the European Union which is the driving force among regulatory authorities in implementing the EU's groundbreaking chemicals legislation for the benefit of human health and the environment as well as for innovation and competitiveness. | |
Explanation | Use of the information, documents and data from the ECHA website is subject to the terms and conditions of this Legal Notice, and subject to other binding limitations provided for under applicable law, the information, documents and data made available on the ECHA website may be reproduced, distributed and/or used, totally or in part, for non-commercial purposes provided that ECHA is acknowledged as the source: "Source: European Chemicals Agency, http://echa.europa.eu/". Such acknowledgement must be included in each copy of the material. ECHA permits and encourages organisations and individuals to create links to the ECHA website under the following cumulative conditions: Links can only be made to webpages that provide a link to the Legal Notice page. | |
Retrosynthesis Analysis
AI-Powered Synthesis Planning: Our tool employs the Template_relevance Pistachio, Template_relevance Bkms_metabolic, Template_relevance Pistachio_ringbreaker, Template_relevance Reaxys, Template_relevance Reaxys_biocatalysis model, leveraging a vast database of chemical reactions to predict feasible synthetic routes.
One-Step Synthesis Focus: Specifically designed for one-step synthesis, it provides concise and direct routes for your target compounds, streamlining the synthesis process.
Accurate Predictions: Utilizing the extensive PISTACHIO, BKMS_METABOLIC, PISTACHIO_RINGBREAKER, REAXYS, REAXYS_BIOCATALYSIS database, our tool offers high-accuracy predictions, reflecting the latest in chemical research and data.
Strategy Settings
Precursor scoring | Relevance Heuristic |
---|---|
Min. plausibility | 0.01 |
Model | Template_relevance |
Template Set | Pistachio/Bkms_metabolic/Pistachio_ringbreaker/Reaxys/Reaxys_biocatalysis |
Top-N result to add to graph | 6 |
Feasible Synthetic Routes
Q1: What is the basic structure of 4,6-decadiyne and where has it been found in nature?
A1: 4,6-Decadiyne is an organic compound characterized by a linear chain of ten carbon atoms containing two conjugated triple bonds between carbons 4-5 and 6-7. It serves as a structural basis for various derivatives. One naturally occurring derivative, (3S,8S)-4,6-decadiyne-1,3,8-triol, has been isolated from the hallucinogenic mushroom Gymnopilus spectabilis [, ].
Q2: Can you provide details on the absolute configuration and synthesis of the naturally occurring 4,6-decadiyne-1,3,8-triol?
A2: The absolute configuration of this compound, isolated from Gymnopilus spectabilis, has been determined to be (3S,8S). This determination was achieved by comparing degradation products of the naturally isolated compound with those of synthetically produced versions [, , ]. Further research led to the first enantiocontrolled synthesis of (3S,8S)-(-)-4,6-decadiyne-1,3,8-triol [, ].
Q3: How does the structure of 4,6-decadiyne derivatives influence their ability to undergo topochemical polymerization?
A3: The arrangement of 4,6-decadiyne units within a crystal lattice is crucial for successful topochemical polymerization. Studies using analogues of 1,6-bis(diphenylamino)-2,4-hexadiyne (THD) showed that subtle structural modifications significantly affect polymerization outcomes. For instance, 1,10-bis(diphenylamino)-4,6-decadiyne (6) exhibited limited polymerization compared to the highly reactive THD. This difference in reactivity was attributed to variations in their crystal packing arrangements, highlighting the importance of structural considerations in designing polymerizable diacetylenes [].
Q4: What unique optical properties are associated with polydiacetylenes, particularly those derived from 4,6-decadiyne?
A4: Polydiacetylenes are known for their intense color changes linked to structural alterations. One study focusing on partially polymerized 4,6-decadiyne-1,10-bis(propylurethane) revealed that these color shifts are associated with solid-phase transitions within the material. Furthermore, the temperature at which these color changes occur can be manipulated by combining different polydiacetylene derivatives, opening possibilities for applications in color-changing materials [].
Q5: Can you elaborate on the electronic properties and potential applications of poly-4,6-decadiyne-1,10-diol-bis(n-butoxycarbonyl-methylurethane) (PDA-3BCMU)?
A5: PDA-3BCMU is a polydiacetylene with intriguing electrical and optical characteristics. Studies have explored the relationship between its molecular weight and electrical conductivity, demonstrating a correlation between these properties []. Additionally, PDA-3BCMU has been investigated for its nonlinear optical properties. Research using planar waveguides of this polymer demonstrated degenerate four-wave mixing, a nonlinear optical process, highlighting its potential in optical switching and signal processing applications [].
Q6: What insights have been gained from studying the relaxation dynamics of photoexcitations in PDA-3BCMU?
A6: Femtosecond absorption and picosecond luminescence spectroscopies have been employed to investigate the behavior of photoexcitations in PDA-3BCMU. These studies revealed that photogenerated excitons rapidly relax to a self-trapped state, influenced by the bulky side chains of the polymer. Furthermore, the decay kinetics of these self-trapped excitons provided insights into the potential energy landscape governing their relaxation processes [].
Q7: Have any specific applications been explored for 1,10-bis(diphenylamino)-4,6-decadiyne beyond its use in polymerization studies?
A7: Yes, 1,10-bis(diphenylamino)-4,6-decadiyne has been investigated as an active component in radiochromic film dosimeters []. These dosimeters are used to measure radiation doses, and the inclusion of this specific diacetylene derivative may offer advantages in terms of sensitivity and response to radiation exposure.
Q8: How has the incorporation of iodine influenced the properties and potential applications of 4,6-decadiyne-based materials?
A8: The introduction of iodine, specifically in the form of I- or I3- ions, into 4,6-decadiyne amphiphiles has opened avenues for creating thin-film polarizers []. These modified amphiphiles form lyotropic liquid crystal phases, which, upon topochemical polymerization, yield films with anisotropic optical properties. The iodine-containing polydiacetylene films exhibit broadband polarization, absorbing light in a specific direction, making them attractive candidates for flexible display technologies.
Q9: What other functional groups have been incorporated into 4,6-decadiyne amphiphiles, and what novel properties have emerged?
A9: Researchers have explored the incorporation of imidazolium groups into 4,6-decadiyne amphiphiles, leading to the creation of stimuli-responsive "secret inks" []. These inks exhibit reversible color and transparency changes in response to external stimuli like temperature and polarized light. These properties stem from the self-assembly and topochemical polymerization behavior of the imidazolium-functionalized diacetylenes, making them promising candidates for applications in security inks, sensors, and displays.
Q10: Beyond Gymnopilus spectabilis, have any other natural sources of 4,6-decadiyne derivatives been identified?
A10: Yes, 4,6-decadiyne glycosides, such as 8Z-decaene-4,6-diyne-1-O-β-D-glucopyranoside and 4,6-decadiyne-1-O-β-D-glucopyranoside, have been isolated from the flowers of Carthamus tinctorius (safflower) [, ]. These findings highlight the presence of 4,6-decadiyne derivatives in diverse plant species and suggest a broader distribution in nature.
Q11: What antioxidant properties have been associated with 4,6-decadiyne derivatives, and which compounds have shown the most promising activity?
A11: Studies investigating the antioxidant potential of compounds isolated from Carthamus tinctorius have identified several 4,6-decadiyne derivatives, including 8Z-decaene-4,6-diyne-1-O-β-D-glucopyranoside and 4,6-decadiyne-1-O-β-D-glucopyranoside, as possessing notable radical scavenging abilities []. These compounds exhibited activity in DPPH assays, suggesting their potential to neutralize harmful free radicals.
Q12: Have any studies explored the conformational changes of water-soluble 4,6-decadiyne-based polymers?
A12: Yes, the conformational transition of the potassium salt of poly[4,6-decadiyne-1,10-diol bis([carboxymethyl]urethane)], a water-soluble polydiacetylene, has been investigated []. By employing techniques such as visible absorption spectroscopy, potentiometric titration, and viscosity measurements, researchers have gained insights into how this polymer's conformation changes in aqueous solutions under varying conditions.
Q13: Are there any known instances of 4,6-decadiyne derivatives exhibiting cytotoxic activity?
A13: Yes, repandiol, a diepoxide derivative of 4,6-decadiyne, has been isolated from the mushrooms Hydnum repandum and H. repandum var. album and found to possess potent cytotoxic activity against various tumor cell lines []. The structure of repandiol, elucidated as (2R,3R,8R,9R)-4,6-decadiyne-2,3:8,9-diepoxy-1,10-diol, highlights the potential of modified 4,6-decadiyne scaffolds in developing anticancer agents.
Q14: Have frustrated Lewis pairs been utilized to modify the 4,6-decadiyne framework, and if so, what are the outcomes?
A14: Research has demonstrated that frustrated Lewis pairs (FLPs), specifically B(C6F5)3/P(o-tolyl)3 (4a) and B(C6F5)3/P(t)Bu3 (4b), react differently with 4,6-decadiyne []. While 4a yields the trans-1,2-addition product, 4b leads to the formation of the trans-1,4-adduct. These findings underscore the potential of FLP chemistry in selectively functionalizing the 4,6-decadiyne skeleton, potentially opening up new avenues for creating novel derivatives.
Avertissement et informations sur les produits de recherche in vitro
Veuillez noter que tous les articles et informations sur les produits présentés sur BenchChem sont destinés uniquement à des fins informatives. Les produits disponibles à l'achat sur BenchChem sont spécifiquement conçus pour des études in vitro, qui sont réalisées en dehors des organismes vivants. Les études in vitro, dérivées du terme latin "in verre", impliquent des expériences réalisées dans des environnements de laboratoire contrôlés à l'aide de cellules ou de tissus. Il est important de noter que ces produits ne sont pas classés comme médicaments et n'ont pas reçu l'approbation de la FDA pour la prévention, le traitement ou la guérison de toute condition médicale, affection ou maladie. Nous devons souligner que toute forme d'introduction corporelle de ces produits chez les humains ou les animaux est strictement interdite par la loi. Il est essentiel de respecter ces directives pour assurer la conformité aux normes légales et éthiques en matière de recherche et d'expérimentation.