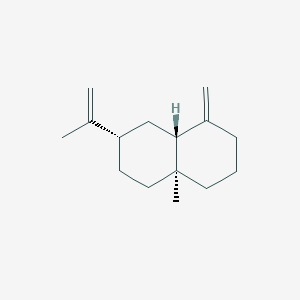
beta-Selinene
- Cliquez sur DEMANDE RAPIDE pour recevoir un devis de notre équipe d'experts.
- Avec des produits de qualité à un prix COMPÉTITIF, vous pouvez vous concentrer davantage sur votre recherche.
Vue d'ensemble
Description
Synthesis Analysis
The synthesis of related compounds, such as beta-seleno amides, has been reported using a ring-opening reaction of chiral 2-oxazolines by selenium nucleophiles . This method has been shown to be efficient and yields products containing various functional groups like thioether, alcohol, and ether. Although this synthesis pertains to beta-seleno amides and not directly to (-)-beta-Selinene, the methodology could potentially be adapted for the synthesis of various seleno-containing organic compounds, which may include sesquiterpenes like (-)-beta-Selinene.
Molecular Structure Analysis
The molecular structure of (-)-beta-Selinene would be expected to contain a complex arrangement of carbon atoms typical of sesquiterpenes. The specific structure would likely include multiple rings and perhaps a chiral center, given the prefix "(-)" indicating optical activity. The synthesis of chiral beta-seleno amides suggests that chiral centers are an important feature in related compounds, which could also be relevant to the structure of (-)-beta-Selinene.
Chemical Reactions Analysis
The provided papers do not detail chemical reactions specific to (-)-beta-Selinene. However, the synthesis of beta-seleno amides and their application in palladium-catalyzed asymmetric allylic alkylation indicates that seleno-containing compounds can participate in complex chemical reactions, leading to products with high enantiomeric excess (up to 98% ee). This suggests that (-)-beta-Selinene, if it contains selenium, could also be involved in similar enantioselective reactions.
Physical and Chemical Properties Analysis
The physical and chemical properties of (-)-beta-Selinene are not described in the provided papers. However, in general, sesquiterpenes are known to be hydrophobic, volatile, and often exhibit optical activity due to the presence of chiral centers. They can have various biological activities and can be found in the essential oils of plants. Betaine, a compound mentioned in one of the papers , is known for its osmoprotective properties, which could be a characteristic of interest if (-)-beta-Selinene has similar properties or if it is used in conjunction with betaine in a biological context.
Applications De Recherche Scientifique
Biotransformation Using Plant Pathogenic Fungus
- The sesquiterpenoid β-selinene can be biotransformed using the plant pathogenic fungus Glomerella cingulata. This process involves the oxidation of β-selinene at specific positions, leading to the production of trihydroxy-β-selinene (Miyazawa, Honjo, & Kameoka, 1997).
Role in Plant Stress Tolerance
- Glycine betaine (GB) and proline, two major osmolytes that accumulate in response to environmental stresses, show a positive relationship with plant stress tolerance. Although not directly mentioned, compounds like β-selinene may play a role in this process (Ashraf & Foolad, 2007).
Identification as Potential Anticancer Agent
- GC-MS analysis of ethanol extract of Zodia leaves identified several compounds, including delta-Selinene, which show potential as anticancer agents. These compounds target breast, lung, and ovarian cancer (Sanora, Mastura, Handoyo, & Purnama, 2019).
Insect Herbivore Defense
- The sesquiterpene hydrocarbons like α- and β-selinene in Hymenaea leaf resins display inhibitory effects on generalist herbivores, such as the beet armyworm. This indicates a potential defensive role against insect predation (Langenheim, Foster, & McGinley, 1980).
Cotton Plant Oil Composition
- In Gossypium hirsutum, alpha- and beta-selinene are major components of its oil, indicating a role in the chemical diversity of this species (Williams et al., 1995).
Maize Defense Against Fungal Pathogens
- Maize terpene synthase21, which produces β-selinene, plays a critical role in antifungal defenses. This enzyme is involved in the biosynthesis of nonvolatile antibiotics like β-costic acid, promoting fungal pathogen resistance (Ding et al., 2017).
Propriétés
{ "Design of the Synthesis Pathway": "The synthesis pathway of (-)-beta-Selinene involves the cyclization of a terpene precursor, which can be obtained from natural sources or synthesized de novo.", "Starting Materials": [ "Geranyl pyrophosphate", "Sodium borohydride", "Acetic acid", "Methanol", "Sulfuric acid" ], "Reaction": [ "Geranyl pyrophosphate is treated with sodium borohydride to reduce the double bond in the isoprenoid chain.", "The resulting alcohol is then treated with acetic acid to form the corresponding acetate ester.", "The ester is then subjected to acidic hydrolysis using sulfuric acid to yield (-)-beta-Selinene." ] } | |
Numéro CAS |
17066-67-0 |
Formule moléculaire |
C15H24 |
Poids moléculaire |
204.35 g/mol |
Nom IUPAC |
(3R,4aS,8aR)-8a-methyl-5-methylidene-3-prop-1-en-2-yl-1,2,3,4,4a,6,7,8-octahydronaphthalene |
InChI |
InChI=1S/C15H24/c1-11(2)13-7-9-15(4)8-5-6-12(3)14(15)10-13/h13-14H,1,3,5-10H2,2,4H3/t13-,14+,15-/m1/s1 |
Clé InChI |
YOVSPTNQHMDJAG-QLFBSQMISA-N |
SMILES isomérique |
CC(=C)[C@@H]1CC[C@]2(CCCC(=C)[C@@H]2C1)C |
SMILES |
CC(=C)C1CCC2(CCCC(=C)C2C1)C |
SMILES canonique |
CC(=C)C1CCC2(CCCC(=C)C2C1)C |
Synonymes |
eta-selinene beta-selinene, ((4aalpha,7alpha,8abeta)-(+-))-isomer beta-selinene, (4aR-(4aalpha,7beta,8aalpha))-isomer beta-selinene, (4aS-(4aalpha,7beta,8abeta))-isome |
Origine du produit |
United States |
Retrosynthesis Analysis
AI-Powered Synthesis Planning: Our tool employs the Template_relevance Pistachio, Template_relevance Bkms_metabolic, Template_relevance Pistachio_ringbreaker, Template_relevance Reaxys, Template_relevance Reaxys_biocatalysis model, leveraging a vast database of chemical reactions to predict feasible synthetic routes.
One-Step Synthesis Focus: Specifically designed for one-step synthesis, it provides concise and direct routes for your target compounds, streamlining the synthesis process.
Accurate Predictions: Utilizing the extensive PISTACHIO, BKMS_METABOLIC, PISTACHIO_RINGBREAKER, REAXYS, REAXYS_BIOCATALYSIS database, our tool offers high-accuracy predictions, reflecting the latest in chemical research and data.
Strategy Settings
Precursor scoring | Relevance Heuristic |
---|---|
Min. plausibility | 0.01 |
Model | Template_relevance |
Template Set | Pistachio/Bkms_metabolic/Pistachio_ringbreaker/Reaxys/Reaxys_biocatalysis |
Top-N result to add to graph | 6 |
Feasible Synthetic Routes
Q & A
Q1: What is the molecular formula and weight of (-)-beta-Selinene?
A1: (-)-beta-Selinene has a molecular formula of C15H24 and a molecular weight of 204.35 g/mol.
Q2: What spectroscopic data is available for the characterization of (-)-beta-Selinene?
A2: (-)-beta-Selinene has been characterized using techniques such as Gas Chromatography-Mass Spectrometry (GC-MS) [, , , , , , , , , , , , , , , , , , , , , , , , , , , , , ], Nuclear Magnetic Resonance (NMR) spectroscopy [, ], and Infrared (IR) spectroscopy []. These techniques provide information about its structure, purity, and presence in various plant extracts.
Q3: What are the key chemical properties of (-)-beta-Selinene?
A3: (-)-beta-Selinene is a colorless liquid at room temperature with a characteristic woody and spicy odor. It is a volatile compound, which contributes to its presence in essential oils extracted from plants.
Q4: How stable is (-)-beta-Selinene under various conditions?
A4: Research suggests that (-)-beta-Selinene can undergo degradation during storage, especially at higher temperatures. [] Further studies are needed to fully understand its stability profile under different conditions such as light exposure, pH, and presence of other chemicals.
Q5: What are the potential applications of (-)-beta-Selinene?
A5: Due to its pleasant aroma, (-)-beta-Selinene might be utilized in fragrance compositions for perfumes, cosmetics, and household products. Additionally, its potential biological activities, such as insecticidal properties [], suggest possible applications in pest control and agriculture.
Q6: What biological activities have been reported for (-)-beta-Selinene?
A6: Studies have shown that (-)-beta-Selinene exhibits insecticidal activity against grain storage insects like Sitophilus zeamais and Tribolium castaneum. [] Additionally, research suggests it might possess anti-atherogenic properties, potentially contributing to its traditional use in managing cardiovascular diseases. []
Q7: Have computational methods been used to study (-)-beta-Selinene?
A8: Yes, computational chemistry tools, such as molecular docking, have been employed to investigate the interactions of (-)-beta-Selinene with biological targets. For instance, molecular docking studies suggest that it can bind to the EmrD efflux pump of Escherichia coli, potentially inhibiting its activity and combating multidrug resistance. []
Q8: Are there any Structure-Activity Relationship (SAR) studies available for (-)-beta-Selinene?
A9: While specific SAR studies focusing solely on (-)-beta-Selinene might be limited, research on related sesquiterpenes provides insights into how structural modifications can influence biological activity. For example, studies on aristolochene synthase, an enzyme involved in the biosynthesis of various sesquiterpenes including (-)-beta-Selinene, highlight the impact of specific amino acid residues on product selectivity and the formation of different sesquiterpene structures. [, ]
Q9: Are there any sustainable practices for the production and utilization of (-)-beta-Selinene?
A11: Exploring eco-friendly extraction methods, such as supercritical fluid extraction using carbon dioxide, could minimize the environmental footprint associated with isolating (-)-beta-Selinene from natural sources. [] Additionally, developing efficient synthetic routes could offer a sustainable alternative to relying solely on natural extraction.
Avertissement et informations sur les produits de recherche in vitro
Veuillez noter que tous les articles et informations sur les produits présentés sur BenchChem sont destinés uniquement à des fins informatives. Les produits disponibles à l'achat sur BenchChem sont spécifiquement conçus pour des études in vitro, qui sont réalisées en dehors des organismes vivants. Les études in vitro, dérivées du terme latin "in verre", impliquent des expériences réalisées dans des environnements de laboratoire contrôlés à l'aide de cellules ou de tissus. Il est important de noter que ces produits ne sont pas classés comme médicaments et n'ont pas reçu l'approbation de la FDA pour la prévention, le traitement ou la guérison de toute condition médicale, affection ou maladie. Nous devons souligner que toute forme d'introduction corporelle de ces produits chez les humains ou les animaux est strictement interdite par la loi. Il est essentiel de respecter ces directives pour assurer la conformité aux normes légales et éthiques en matière de recherche et d'expérimentation.