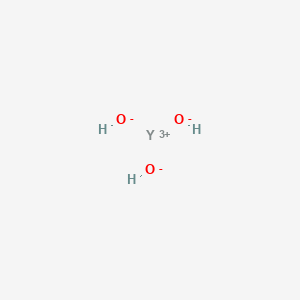
Yttrium trihydroxide
- Cliquez sur DEMANDE RAPIDE pour recevoir un devis de notre équipe d'experts.
- Avec des produits de qualité à un prix COMPÉTITIF, vous pouvez vous concentrer davantage sur votre recherche.
Vue d'ensemble
Description
Yttrium trihydroxide (Y(OH)₃·H₂O), also known as yttrium(III) hydroxide, is a rare earth hydroxide with applications in catalysis, ceramics, and advanced material synthesis . Its chemical identifiers include CAS numbers 12688-14-1 and 1311-34-8, a PubChem CID of 85438, and the IUPAC name yttrium(3+) trihydroxide .
This compound is synthesized via precipitation reactions, such as the transformation of yttrium hydroxide into yttrium citrate through reactions with citric acid or its salts . Its solubility behavior in aqueous systems is critical for optimizing synthesis conditions, as demonstrated by solubility diagrams for yttrium hydroxide and yttrium citrate .
Méthodes De Préparation
Precipitation Methods
Precipitation remains the most accessible route for Y(OH)₃ synthesis, offering scalability and minimal infrastructure requirements.
Simple Aqueous Precipitation
The reaction between yttrium nitrate (Y(NO₃)₃) and sodium hydroxide (NaOH) in aqueous media yields amorphous Y(OH)₃ as a gelatinous precipitate . Stoichiometric control ensures complete precipitation:
Y(NO₃)₃ + 3 NaOH → Y(OH)₃↓ + 3 NaNO₃
Adjusting the NaOH concentration (pH ~8.4–13) influences colloidal stability, with higher alkalinity favoring crystalline phases . For instance, a 3.33:1 molar ratio of NaOH:YCl₃ produces phase-pure Y(OH)₃ nanoparticles (40–50 nm) .
Controlled pH Precipitation
A two-step alkali addition strategy enhances morphological uniformity. Initial partial neutralization of YCl₃ to pH 6.8 followed by gradual NaOH titration to pH 13 fosters anisotropic growth of hexagonal Y(OH)₃ nanorods (diameter: 80–120 nm, length: 1–2 μm) . This method mitigates rapid nucleation, reducing agglomeration.
Additive-Assisted Precipitation
Surfactants like ethylenediaminetetraacetic acid (EDTA) or polyethyleneimine (PEI) template specific morphologies. For example, 0.1 M EDTA in Y(NO₃)₃/NaOH systems yields monodisperse Y(OH)₃ nanoflowers (300–400 nm) . Additives adsorb onto crystal facets, modulating growth kinetics and surface energy disparities.
Table 1: Precipitation Method Parameters and Outcomes
Hydrothermal and Solvothermal Synthesis
Hydrothermal techniques enable precise crystallographic control, leveraging elevated temperatures and pressures.
Hydrothermal Parameters and Morphology Control
Reacting Y(NO₃)₃·6H₂O with NaOH (3–6 M) at 200–220°C for 8–24 hours produces Y(OH)₃ nanotubes (diameter: 50–100 nm, length: 1–5 μm) . Prolonged reaction times (>12 hours) at pH 11–12 favor Ostwald ripening, transitioning nanoparticles to microrods . For instance, 12-hour hydrothermal treatment at 180°C yields monophasic Y(OH)₃ nanobars (200 nm width) .
Solvent Effects in Solvothermal Synthesis
Non-aqueous media like methanol or ethanol alter nucleation dynamics. A solvothermal reaction of YCl₃ in methanol with urea (200°C, 2.5 hours) generates layered Y(OH)₃ nanosheets (thickness: 10–20 nm) . Ethanol’s lower dielectric constant reduces ion mobility, favoring 2D growth over isotropic particle formation.
Table 2: Hydrothermal/Solvothermal Synthesis Conditions
Precursor | Solvent | Temp (°C) | Time (h) | pH | Product | Size | Source |
---|---|---|---|---|---|---|---|
Y(NO₃)₃·6H₂O | H₂O | 220 | 24 | 11–12 | Nanotubes | 50 nm × 1 μm | |
YCl₃ | Methanol | 200 | 2.5 | – | Layered nanosheets | 10–20 nm thick | |
Y(OH)CO₃ | H₂O | 190 | 48 | 13 | Nanorods | 30 nm × 500 nm |
Electrodeposition Techniques
Cathodic electrodeposition provides a room-temperature route for Y(OH)₃ thin films. Applying −1.2 V (vs. Ag/AgCl) to a stainless steel electrode in Y(NO₃)₃ solution (0.1 M, pH 5) deposits adherent Y(OH)₃ nanoplatelets (thickness: 20–30 nm) . Post-annealing at 600°C converts these films to porous Y₂O₃, retaining nanostructural integrity.
Comparative Analysis of Synthesis Methods
Table 3: Methodological Advantages and Limitations
Method | Advantages | Limitations | Scale-Up Potential |
---|---|---|---|
Precipitation | Low cost, rapid synthesis | Broad size distribution | High |
Hydrothermal | Crystalline control, diverse morphologies | High energy input | Moderate |
Electrodeposition | Room-temperature, thin films | Limited to conductive substrates | Low |
Analyse Des Réactions Chimiques
Types of Reactions: Yttrium trihydroxide undergoes several types of chemical reactions, including:
Reaction with Acids: As an alkali, this compound reacts readily with acids to form yttrium salts and water. For example: [ \text{Y(OH)}_3 + 3 \text{HNO}_3 \rightarrow \text{Y(NO}_3\text{)}_3 + 3 \text{H}_2\text{O} ] [ 2 \text{Y(OH)}_3 + 3 \text{H}_2\text{SO}_4 \rightarrow \text{Y}_2(\text{SO}_4)_3 + 6 \text{H}_2\text{O} ]
Decomposition: When heated, this compound decomposes to form yttrium oxide and water: [ 2 \text{Y(OH)}_3 \rightarrow \text{Y}_2\text{O}_3 + 3 \text{H}_2\text{O} ]
Common Reagents and Conditions:
Acids: Nitric acid and sulfuric acid are commonly used to react with this compound.
Heat: Decomposition requires heating the compound to high temperatures.
Major Products:
Yttrium Nitrate: Formed when reacting with nitric acid.
Yttrium Sulfate: Formed when reacting with sulfuric acid.
Yttrium Oxide: Formed upon decomposition by heating.
Applications De Recherche Scientifique
Material Science Applications
Yttrium trihydroxide is primarily utilized in the development of advanced materials due to its high thermal stability and chemical resistance.
- Ceramics and Glasses : Yttrium hydroxide is a precursor for yttria-stabilized zirconia (YSZ), which is used in high-temperature applications such as thermal barrier coatings and solid oxide fuel cells. The incorporation of yttrium enhances the mechanical strength and thermal stability of ceramic materials .
- Phosphors : this compound serves as a host material for rare-earth dopants, which are critical in the production of phosphors for LED lighting and display technologies. For instance, Y(OH)₃ doped with europium (Eu) is widely used in red phosphors for various lighting applications .
Biomedical Applications
This compound has garnered attention in the biomedical field, particularly for its potential in drug delivery systems and imaging.
- Drug Delivery Systems : Researchers are exploring yttrium-based nanoparticles for targeted drug delivery. The unique properties of yttrium compounds allow for the encapsulation of therapeutic agents, enabling controlled release at specific sites within the body .
- Biosensors : Yttrium hydroxide nanoparticles are being investigated for use in biosensors due to their biocompatibility and ability to enhance signal detection through luminescence .
- Antimicrobial Activity : Studies have shown that yttrium hydroxide exhibits moderate antibacterial properties, making it a candidate for antimicrobial coatings in medical devices .
Electronics and Optoelectronics
The electronic properties of this compound make it suitable for various electronic applications.
- Capacitors and Resistors : Yttrium hydroxide can be used in the fabrication of capacitors and resistors due to its dielectric properties, contributing to the development of high-performance electronic components .
- Optical Devices : As a luminescent material, this compound is employed in optical devices such as lasers and scintillators. Its ability to emit light when excited makes it valuable for applications in photonics .
Case Study 1: Biomedical Imaging
A study investigated the use of Y(OH)₃ nanoparticles as contrast agents in imaging techniques. Results demonstrated enhanced imaging quality due to their luminescent properties when doped with specific rare earth ions .
Case Study 2: Antimicrobial Coatings
Research focused on incorporating yttrium hydroxide into polymer matrices for medical devices. The coatings exhibited significant antimicrobial activity against common pathogens, suggesting potential for infection prevention .
Mécanisme D'action
The mechanism by which yttrium trihydroxide exerts its effects is primarily through its reactivity with other compounds. In medical applications, yttrium-90 derived from this compound emits beta radiation, which is used to target and destroy cancer cells. The molecular targets and pathways involved include the direct interaction of beta particles with cellular DNA, leading to cell death .
Comparaison Avec Des Composés Similaires
Comparison with Similar Yttrium Compounds
The following analysis compares yttrium trihydroxide with other yttrium-based compounds in terms of chemical structure, solubility, applications, and safety profiles.
Chemical and Structural Properties
Key Observations :
- This compound is distinct in its hydroxide ligand structure, whereas chloride, acetate, and citrate derivatives feature halide or carboxylate ligands.
- Hydration states vary: yttrium chloride and acetate often exist as hydrates (e.g., YCl₃·xH₂O ), while yttrium trihydride is anhydrous .
Solubility and Reactivity
- This compound : Exhibits low solubility in water, necessitating acidic conditions (e.g., citric acid) for dissolution and further synthesis of complexes like yttrium citrate .
- Yttrium Chloride : Highly water-soluble (anhydrous: 82.6 g/100 mL at 20°C), enabling its use in aqueous-phase reactions .
- Yttrium Acetate : Moderately soluble in polar solvents; used in sol-gel processes for ceramic materials .
- Yttrium Citrate : Forms stable complexes in solution, with solubility influenced by pH and ligand concentration .
Research Findings and Gaps
- Synthesis Optimization : this compound’s conversion to citrate complexes is pH-dependent, with optimal yields achieved at pH 4–6 .
- Toxicity Data: Limited studies on this compound’s chronic toxicity exist; most safety data focus on chloride and nitrate salts .
- Industrial Use : Yttrium trihydride’s hydrogen storage capacity is under investigation for clean energy applications, contrasting with trihydroxide’s role in ceramics .
Activité Biologique
Yttrium trihydroxide (Y(OH)₃) is a compound of yttrium that has garnered attention in various fields, particularly in biomedical applications due to its unique properties. This article explores the biological activity of this compound, focusing on its antibacterial, anticancer, and neuroprotective effects, as well as its potential applications in tissue engineering.
1. Antibacterial Activity
This compound exhibits significant antibacterial properties. Research indicates that it can suppress the growth of both gram-positive and gram-negative bacteria. The mechanism of action involves the penetration of yttrium hydroxide nanoparticles into bacterial cells, leading to the generation of reactive oxygen species (ROS), which ultimately results in bacterial cell death.
Table 1: Antibacterial Efficacy of this compound Nanoparticles
Bacterial Strain | Minimum Inhibitory Concentration (MIC) |
---|---|
Escherichia coli | 11 µg/mL |
Pseudomonas aeruginosa | 10 µg/mL |
Staphylococcus aureus | 13 µg/mL |
Salmonella marcens | 13 µg/mL |
The above table summarizes the effective concentrations required to inhibit the growth of various bacterial strains using this compound nanoparticles, showcasing its potential as an antibacterial agent .
2. Anticancer Activity
This compound has also been investigated for its anticancer properties. Studies have shown that when synthesized with plant extracts, it exhibits selective toxicity towards cancer cells while sparing normal cells. For example, in experiments involving kidney cancer cell lines such as Madin-Darby Canine Kidney (MDCK) and Caki-2, this compound demonstrated a flake-like morphology with a size of approximately 11 nm, which correlated with its cytotoxic effects on cancer cells at higher concentrations without affecting normal cells .
Case Study: Cytotoxic Effects on Cancer Cell Lines
In a controlled study, this compound nanoparticles were administered to MDCK and Caki-2 cell lines. The results indicated:
- Caki-2 Cells : Significant reduction in cell viability at concentrations above 50 µg/mL.
- MDCK Cells : Minimal toxicity observed even at high concentrations.
These findings suggest that this compound could be utilized as a targeted therapeutic agent in cancer treatment protocols .
3. Neuroprotective Effects
Recent studies have highlighted the neuroprotective properties of this compound nanoparticles. In animal models, administration of these nanoparticles was associated with reduced oxidative stress markers and inflammation in brain tissues. The compound appears to mitigate damage induced by other nanoparticles such as calcium hydroxide (Ca(OH)₂) and calcium titanate (CaTiO₃), which are known to generate ROS and cause genomic DNA damage.
Table 2: Neuroprotective Effects of this compound
Treatment Group | Genomic DNA Damage (%) | Mitochondrial Membrane Integrity (%) |
---|---|---|
Control Group | 5 | 90 |
Ca(OH)₂ Alone | 45 | 40 |
CaTiO₃ Alone | 30 | 60 |
Y(OH)₃ Alone | 6 | 88 |
Y(OH)₃ + Ca(OH)₂ + CaTiO₃ Combined | 10 | 85 |
The data indicates that this compound not only protects against ROS-induced damage but also helps maintain mitochondrial integrity when co-administered with harmful nanoparticles .
4. Applications in Tissue Engineering
This compound has shown promise in tissue engineering applications. When incorporated into polymer scaffolds, it enhances cell proliferation and exhibits angiogenic properties. This is particularly beneficial for applications requiring improved vascularization and cellular integration in engineered tissues.
Summary of Findings
- Cell Proliferation : Enhanced when Y(OH)₃ is embedded in polycaprolactone (PCL) scaffolds.
- Angiogenesis : Promotes blood vessel formation, crucial for tissue regeneration.
Q & A
Basic Research Questions
Q. What are the standard synthesis protocols for yttrium trihydroxide (Y(OH)₃), and how do reaction conditions influence crystallinity and phase purity?
- Methodological Answer: this compound is typically synthesized via hydrothermal or precipitation methods. For example, alkaline hydrolysis of yttrium nitrate hexahydrate (Y(NO₃)₃·6H₂O) under controlled pH (9–11) and temperature (80–120°C) yields Y(OH)₃ nanoparticles. Phase purity can be verified using X-ray diffraction (XRD) to confirm the absence of secondary phases like YO(OH) or Y₂O₃. Adjusting reaction time and temperature impacts particle size distribution, as shown in studies comparing hydrothermal vs. room-temperature precipitation .
Q. Which characterization techniques are most effective for analyzing the structural and morphological properties of Y(OH)₃?
- Methodological Answer: A combination of XRD (for crystallographic structure), thermogravimetric analysis (TGA, to study dehydration pathways), and scanning electron microscopy (SEM) is recommended. For nanoscale particles, dynamic light scattering (DLS) and BET surface area analysis provide insights into colloidal stability and surface reactivity. Cross-referencing TGA data with differential scanning calorimetry (DSC) helps identify decomposition intermediates, critical for reproducibility .
Advanced Research Questions
Q. How can researchers resolve contradictions in reported thermal decomposition pathways of Y(OH)₃ to Y₂O₃?
- Methodological Answer: Discrepancies in decomposition temperatures (ranging from 300°C to 600°C) often arise from differences in precursor purity, heating rates, or atmospheric conditions (e.g., inert vs. oxidizing environments). To address this, replicate experiments using standardized conditions (e.g., 5°C/min heating rate under argon) and employ in-situ XRD or Fourier-transform infrared spectroscopy (FTIR) to track phase transitions dynamically. Comparative studies using Y(OH)₃ samples with varying hydroxyl group densities (via controlled synthesis) can isolate key variables .
Q. What computational models are suitable for predicting the electronic structure and catalytic potential of Y(OH)₃ surfaces?
- Methodological Answer: Density functional theory (DFT) simulations, particularly using hybrid functionals (e.g., HSE06), can model surface hydroxylation effects and bandgap variations. Pairing DFT with ab initio molecular dynamics (AIMD) helps simulate hydration dynamics under realistic conditions. Validate models against experimental X-ray photoelectron spectroscopy (XPS) data for surface composition and valence band alignment .
Q. How do lattice defects in Y(OH)₃ influence its application in photocatalysis or ion-exchange processes?
- Methodological Answer: Introduce controlled defects (e.g., oxygen vacancies via calcination or doping with transition metals) and evaluate their impact using positron annihilation spectroscopy (PAS) for vacancy detection. Electrochemical impedance spectroscopy (EIS) and transient absorption spectroscopy (TAS) quantify charge-carrier lifetimes and mobility. Cross-disciplinary approaches, such as coupling defect engineering with operando Raman spectroscopy, reveal structure-activity relationships .
Q. What strategies improve the reproducibility of Y(OH)₃-based composite materials for environmental remediation?
- Methodological Answer: Standardize precursor mixing ratios (e.g., Y(OH)₃ with graphene oxide) and employ sol-gel or atomic layer deposition (ALD) for uniform coatings. Use synchrotron-based X-ray absorption near-edge structure (XANES) to assess interfacial bonding. Reproducibility challenges often stem from inconsistent colloidal stability; address this by optimizing surfactant concentrations (e.g., cetyltrimethylammonium bromide, CTAB) during synthesis .
Q. Data Management and Validation
Q. How should researchers handle conflicting spectroscopic data when characterizing Y(OH)₃ hydration states?
- Methodological Answer: Combine multiple techniques: FTIR for O-H stretching modes (3200–3600 cm⁻¹), nuclear magnetic resonance (NMR) for proton environments, and quasi-elastic neutron scattering (QENS) for water mobility. Statistical tools like principal component analysis (PCA) can identify outliers in datasets, while machine learning algorithms (e.g., partial least squares regression) correlate spectral features with hydration levels .
Q. What frameworks ensure ethical and transparent reporting of Y(OH)₃ research data?
- Methodological Answer: Adhere to FAIR principles (Findable, Accessible, Interoperable, Reusable). Provide raw XRD/TGA data in repositories like Zenodo, accompanied by detailed metadata (e.g., synthesis conditions, instrument calibration logs). For peer review, include error margins in crystallographic parameters (e.g., lattice constants ±0.02 Å) and specify software versions (e.g., TOPAS v6.0 for Rietveld refinement) .
Q. Cross-Disciplinary Applications
Q. Can Y(OH)₃ serve as a precursor for high-temperature superconductors or advanced ceramics?
- Methodological Answer: Y(OH)₃-derived Y₂O₃ is a critical precursor for yttrium-stabilized zirconia (YSZ) and yttrium barium copper oxide (YBCO) superconductors. Optimize calcination protocols to control Y₂O₃ particle size (<50 nm preferred for sintering). Pair with spark plasma sintering (SPS) for dense ceramics. Recent studies on yttrium hydrides (e.g., YH₆) suggest analogous pathways for high-pressure synthesis of novel materials .
Propriétés
Numéro CAS |
16469-22-0 |
---|---|
Formule moléculaire |
H6O3Y |
Poids moléculaire |
142.952 g/mol |
Nom IUPAC |
yttrium;trihydrate |
InChI |
InChI=1S/3H2O.Y/h3*1H2; |
Clé InChI |
GFDKELMFCRQUSG-UHFFFAOYSA-N |
SMILES |
[OH-].[OH-].[OH-].[Y+3] |
SMILES canonique |
O.O.O.[Y] |
Key on ui other cas no. |
16469-22-0 |
Origine du produit |
United States |
Retrosynthesis Analysis
AI-Powered Synthesis Planning: Our tool employs the Template_relevance Pistachio, Template_relevance Bkms_metabolic, Template_relevance Pistachio_ringbreaker, Template_relevance Reaxys, Template_relevance Reaxys_biocatalysis model, leveraging a vast database of chemical reactions to predict feasible synthetic routes.
One-Step Synthesis Focus: Specifically designed for one-step synthesis, it provides concise and direct routes for your target compounds, streamlining the synthesis process.
Accurate Predictions: Utilizing the extensive PISTACHIO, BKMS_METABOLIC, PISTACHIO_RINGBREAKER, REAXYS, REAXYS_BIOCATALYSIS database, our tool offers high-accuracy predictions, reflecting the latest in chemical research and data.
Strategy Settings
Precursor scoring | Relevance Heuristic |
---|---|
Min. plausibility | 0.01 |
Model | Template_relevance |
Template Set | Pistachio/Bkms_metabolic/Pistachio_ringbreaker/Reaxys/Reaxys_biocatalysis |
Top-N result to add to graph | 6 |
Feasible Synthetic Routes
Avertissement et informations sur les produits de recherche in vitro
Veuillez noter que tous les articles et informations sur les produits présentés sur BenchChem sont destinés uniquement à des fins informatives. Les produits disponibles à l'achat sur BenchChem sont spécifiquement conçus pour des études in vitro, qui sont réalisées en dehors des organismes vivants. Les études in vitro, dérivées du terme latin "in verre", impliquent des expériences réalisées dans des environnements de laboratoire contrôlés à l'aide de cellules ou de tissus. Il est important de noter que ces produits ne sont pas classés comme médicaments et n'ont pas reçu l'approbation de la FDA pour la prévention, le traitement ou la guérison de toute condition médicale, affection ou maladie. Nous devons souligner que toute forme d'introduction corporelle de ces produits chez les humains ou les animaux est strictement interdite par la loi. Il est essentiel de respecter ces directives pour assurer la conformité aux normes légales et éthiques en matière de recherche et d'expérimentation.