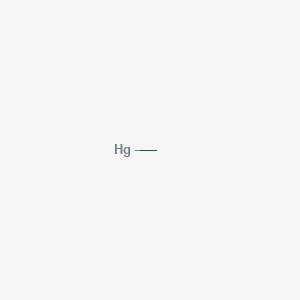
Methylmercury
- Cliquez sur DEMANDE RAPIDE pour recevoir un devis de notre équipe d'experts.
- Avec des produits de qualité à un prix COMPÉTITIF, vous pouvez vous concentrer davantage sur votre recherche.
Vue d'ensemble
Description
Il est hautement résistant à un large spectre de bêta-lactamases et est efficace contre une large gamme d'organismes gram-positifs et gram-négatifs, aérobies et anaérobies . L'Epocelin est couramment utilisé pour traiter les infections bactériennes dans diverses parties du corps, notamment le cerveau, les poumons, l'oreille, les voies urinaires, la peau, les tissus mous, les os, les articulations, le sang et le cœur .
Méthodes De Préparation
Voies de synthèse et conditions réactionnelles : L'Epocelin est synthétisé par une série de réactions chimiques impliquant l'incorporation d'un cycle thiazole et d'un groupe méthoxyimino dans la structure de base de la céphalosporine. La synthèse implique généralement les étapes suivantes :
Formation du cycle thiazole : Le cycle thiazole est formé en faisant réagir un thioamide approprié avec un composé halogéné.
Incorporation du groupe méthoxyimino : Le groupe méthoxyimino est introduit par une réaction avec un dérivé d'oxime approprié.
Couplage avec le noyau de céphalosporine : Les groupes thiazole et méthoxyimino sont ensuite couplés avec le noyau de céphalosporine pour former le composé final.
Méthodes de production industrielle : Dans les milieux industriels, l'Epocelin est produit par des processus de fermentation à grande échelle suivis d'une modification chimique. Le processus de fermentation implique l'utilisation de souches spécifiques de micro-organismes pour produire le noyau de céphalosporine, qui est ensuite modifié chimiquement pour introduire les groupes thiazole et méthoxyimino .
Analyse Des Réactions Chimiques
Types de réactions : L'Epocelin subit diverses réactions chimiques, notamment :
Oxydation : L'Epocelin peut être oxydé pour former des sulfoxydes et des sulfones.
Réduction : Les réactions de réduction peuvent convertir l'Epocelin en ses dérivés alcooliques correspondants.
Substitution : L'Epocelin peut subir des réactions de substitution, en particulier au niveau du cycle thiazole, pour former divers dérivés.
Réactifs et conditions courants :
Oxydation : Les agents oxydants courants comprennent le peroxyde d'hydrogène et le permanganate de potassium.
Réduction : Des agents réducteurs tels que le borohydrure de sodium et l'hydrure de lithium et d'aluminium sont utilisés.
Substitution : Les réactions de substitution impliquent souvent des nucléophiles tels que les amines et les thiols.
Principaux produits formés :
Oxydation : Sulfoxydes et sulfones.
Réduction : Dérivés alcooliques.
Substitution : Divers dérivés thiazoliques.
4. Applications de la recherche scientifique
L'Epocelin a un large éventail d'applications de recherche scientifique, notamment :
Chimie : Utilisé comme composé modèle pour étudier la résistance aux bêta-lactamases et le développement de nouveaux antibiotiques.
Biologie : Employé dans la recherche sur la synthèse de la paroi cellulaire bactérienne et les mécanismes de résistance aux antibiotiques.
Médecine : Utilisé dans des études cliniques pour évaluer son efficacité et sa sécurité dans le traitement de diverses infections bactériennes.
Industrie : Appliqué dans le développement de nouvelles formulations pharmaceutiques et de systèmes d'administration de médicaments .
5. Mécanisme d'action
L'Epocelin exerce ses effets en inhibant la synthèse de la paroi cellulaire bactérienne. Il se lie aux protéines de liaison à la pénicilline (PBP) sur la paroi cellulaire bactérienne, empêchant la réticulation des chaînes de peptidoglycanes, qui est essentielle à l'intégrité de la paroi cellulaire. Cela conduit à l'affaiblissement et à la lyse éventuelle de la cellule bactérienne .
Composés similaires :
- Céfotaxime
- Ceftriaxone
- Céfuroxime
Comparaison : L'Epocelin est unique parmi les céphalosporines en raison de sa forte résistance aux bêta-lactamases et de son large spectre d'activité contre les organismes gram-positifs et gram-négatifs. Contrairement aux autres céphalosporines de troisième génération, la chaîne latérale C-3 de l'Epocelin a été supprimée pour empêcher la désactivation par les enzymes hydrolytiques .
Applications De Recherche Scientifique
Epocelin has a wide range of scientific research applications, including:
Chemistry: Used as a model compound for studying beta-lactamase resistance and the development of new antibiotics.
Biology: Employed in research on bacterial cell wall synthesis and the mechanisms of antibiotic resistance.
Medicine: Used in clinical studies to evaluate its efficacy and safety in treating various bacterial infections.
Industry: Applied in the development of new pharmaceutical formulations and drug delivery systems .
Mécanisme D'action
Epocelin exerts its effects by inhibiting bacterial cell wall synthesis. It binds to penicillin-binding proteins (PBPs) on the bacterial cell wall, preventing the cross-linking of peptidoglycan chains, which is essential for cell wall integrity. This leads to the weakening and eventual lysis of the bacterial cell .
Comparaison Avec Des Composés Similaires
- Cefotaxime
- Ceftriaxone
- Cefuroxime
Comparison: Epocelin is unique among cephalosporins due to its high resistance to beta-lactamases and its broad spectrum of activity against both gram-positive and gram-negative organisms. Unlike other third-generation cephalosporins, Epocelin’s C-3 side chain has been removed to prevent deactivation by hydrolytic enzymes .
Propriétés
Numéro CAS |
16056-34-1 |
---|---|
Formule moléculaire |
CH3Hg |
Poids moléculaire |
215.63 g/mol |
Nom IUPAC |
methylmercury |
InChI |
InChI=1S/CH3.Hg/h1H3; |
Clé InChI |
JJWSNOOGIUMOEE-UHFFFAOYSA-N |
SMILES |
C[Hg] |
SMILES canonique |
C[Hg] |
16056-34-1 | |
Origine du produit |
United States |
Avertissement et informations sur les produits de recherche in vitro
Veuillez noter que tous les articles et informations sur les produits présentés sur BenchChem sont destinés uniquement à des fins informatives. Les produits disponibles à l'achat sur BenchChem sont spécifiquement conçus pour des études in vitro, qui sont réalisées en dehors des organismes vivants. Les études in vitro, dérivées du terme latin "in verre", impliquent des expériences réalisées dans des environnements de laboratoire contrôlés à l'aide de cellules ou de tissus. Il est important de noter que ces produits ne sont pas classés comme médicaments et n'ont pas reçu l'approbation de la FDA pour la prévention, le traitement ou la guérison de toute condition médicale, affection ou maladie. Nous devons souligner que toute forme d'introduction corporelle de ces produits chez les humains ou les animaux est strictement interdite par la loi. Il est essentiel de respecter ces directives pour assurer la conformité aux normes légales et éthiques en matière de recherche et d'expérimentation.