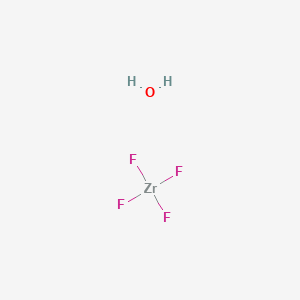
Zirconium(IV) fluoride hydrate
Vue d'ensemble
Description
Zirconium(IV) fluoride hydrate (ZrF₄·xH₂O) is a hydrated metal fluoride compound with the molecular formula H₂F₄OZr and a molecular weight of 185.23 g/mol . It is characterized by its hygroscopic nature and corrosive properties, requiring storage in cool, dry, and airtight conditions to prevent hydrolysis or decomposition . Structurally, it contains zirconium in the +4 oxidation state coordinated by fluoride ions and water molecules, forming a complex lattice .
This compound is widely utilized in environmental and industrial applications. For example, it serves as a precursor for synthesizing fluoride-releasing monomers in dental composites and is incorporated into hybrid sorbents (e.g., zirconium-carbon composites) for efficient fluoride removal from water . Its high affinity for fluoride ions stems from strong Zr–F bonds, making it effective in adsorption processes .
Méthodes De Préparation
Direct Reaction of Zirconium Dioxide with Hydrofluoric Acid
The most widely documented method involves the reaction of zirconium dioxide (ZrO₂) with hydrofluoric acid (HF). This exothermic process proceeds as follows:
2 + 4\text{HF} \rightarrow \text{ZrF}4 + 2\text{H}_2\text{O}
Reaction Conditions :
-
Acid Concentration : Concentrated HF (48–70% w/w) ensures complete conversion. Lower concentrations risk incomplete reaction or ZrO₂ residue .
-
Temperature : Elevated temperatures (80–120°C) accelerate kinetics but require corrosion-resistant reactors (e.g., Hastelloy or PTFE-lined vessels) .
-
Stoichiometry : A molar ratio of 1:4 for ZrO₂:HF is optimal. Excess HF improves yield but complicates purification .
Post-Reaction Processing :
The resultant ZrF₄ is hygroscopic and readily forms hydrates upon exposure to atmospheric moisture. Controlled hydration in desiccators or under nitrogen flow regulates the hydrate stoichiometry (x = 1–3) .
Metallic Zirconium Fluorination
Elemental zirconium reacts vigorously with anhydrous HF gas to form ZrF₄, which is subsequently hydrated:
4 + 2\text{H}2
Key Considerations :
-
Gas Purity : Anhydrous HF (≥99.9%) minimizes oxide impurities. Moisture introduces ZrO₂ contaminants .
-
Temperature Control : Reactions at 200–300°C in nickel reactors prevent passivation. Below 200°C, incomplete fluorination occurs .
-
Safety : H₂ gas generation necessitates inert atmospheres to avoid explosions .
Hydration Protocol :
The anhydrous ZrF₄ product is dissolved in deionized water and recrystallized at 25–40°C to yield the hydrate .
Hydrothermal Synthesis
Hydrothermal methods enable precise control over crystal structure and hydration states. A typical procedure involves:
-
Dissolving ZrO₂ in aqueous HF (10–20% w/w).
-
Sealing the solution in autoclaves at 150–200°C for 12–48 hours.
-
Cooling to room temperature to precipitate ZrF₄·xH₂O crystals .
Advantages :
-
High crystallinity and phase purity.
-
Tunable hydrate stoichiometry via pressure and temperature modulation .
Limitations :
-
High equipment costs and scalability challenges.
Acid Leaching and Crystallization
Adapted from zirconium sulfate production , this method involves:
-
Leaching : Zirconium ores (e.g., zircon sand, ZrSiO₄) are digested in concentrated HF (60–80% w/w) at 80–100°C.
-
Dilution : The leachate is diluted to 45–55% HF, inducing ZrF₄·xH₂O crystallization.
-
Filtration : Crystals are separated via vacuum filtration and washed with ethanol .
Optimization Insights :
-
Acid Concentration : Dilution to ~55% HF maximizes yield by minimizing ZrF₄ solubility .
-
Impurity Removal : Hot filtration at 70–80°C eliminates silica and unreacted ZrSiO₄ .
Comparative Analysis of Preparation Methods
Method | Yield (%) | Purity (%) | Hydrate Control | Scalability |
---|---|---|---|---|
ZrO₂ + HF | 85–92 | 90–95 | Moderate | High |
Metallic Zr Fluorination | 78–88 | 95–98 | High | Moderate |
Hydrothermal | 90–95 | 98–99.5 | Excellent | Low |
Acid Leaching | 80–85 | 85–90 | Low | High |
Key Findings :
-
Hydrothermal Synthesis achieves the highest purity but is impractical for industrial-scale production .
-
Direct ZrO₂ Reaction balances yield and scalability, making it the preferred commercial method .
-
Acid Leaching is cost-effective for low-purity applications (e.g., fluoride adsorption) .
Challenges and Mitigation Strategies
-
Hydrolysis Prevention :
ZrF₄·xH₂O hydrolyzes to ZrO₂ in humid environments. Storage in desiccators with P₂O₅ or under argon mitigates degradation . -
Impurity Control :
Silica (SiO₂) from zircon sand leachates is removed via hot filtration or chelating agents (e.g., EDTA) . -
Corrosion Management :
PTFE-lined reactors and Hastelloy equipment resist HF corrosion .
Industrial Production Workflow
A representative large-scale process involves:
-
Leaching milled zircon sand in 70% HF at 90°C for 6 hours.
-
Diluting to 55% HF and cooling to 25°C to crystallize ZrF₄·3H₂O.
Annual Output : ~5,000 metric tons globally, primarily for optical glass and nuclear applications .
Emerging Techniques
Analyse Des Réactions Chimiques
Types of Reactions
Zirconium(IV) fluoride hydrate undergoes various chemical reactions, including:
Hydrolysis: When exposed to moisture, this compound can hydrolyze to form zirconium hydroxide and hydrofluoric acid.
Complexation: It can form complexes with other fluoride ions or ligands, leading to the formation of various zirconium fluoride complexes.
Reduction: Zirconium(IV) fluoride can be reduced to lower oxidation states of zirconium, although this is less common.
Common Reagents and Conditions
Hydrolysis: Water or moisture is the primary reagent for hydrolysis reactions.
Complexation: Fluoride salts or other ligands can be used to form complexes with this compound.
Reduction: Reducing agents such as hydrogen gas or metals can be used under specific conditions to reduce zirconium(IV) fluoride.
Major Products Formed
Hydrolysis: Zirconium hydroxide and hydrofluoric acid.
Complexation: Various zirconium fluoride complexes.
Reduction: Lower oxidation states of zirconium, such as zirconium(III) fluoride.
Applications De Recherche Scientifique
Materials Science
ZBLAN Glass Production
One of the primary commercial applications of zirconium(IV) fluoride is as a precursor for ZBLAN (Zirconium-Barium-Lanthanum-Aluminum-Sodium) glasses. These fluoride glasses are known for their low refractive index and high transparency in the infrared region, making them suitable for fiber optics and laser applications. The unique properties of ZBLAN glasses arise from the incorporation of zirconium fluoride, which enhances their thermal and mechanical stability .
Fluorozirconate Glasses
Zirconium(IV) fluoride is also a key component in fluorozirconate glasses, which are utilized in optical devices due to their excellent transmission characteristics. These materials are particularly advantageous in applications requiring low phonon energy, such as solid-state lasers and infrared optics .
Chemical Applications
Coolants in Nuclear Reactors
In nuclear engineering, zirconium(IV) fluoride has been employed as a coolant in various reactor designs. For instance, it has been part of mixtures used in the Aircraft Reactor Experiment and the Molten-Salt Reactor Experiment, where it contributes to efficient heat transfer and stability under high-temperature conditions .
Catalysis
Zirconium(IV) fluoride serves as a catalyst in several chemical reactions. Its ability to facilitate reactions involving fluorinated compounds makes it valuable in organic synthesis and material fabrication processes .
Biomedical Applications
Polymeric Calcium Phosphate Cements
Research indicates that adding zirconium(IV) fluoride to polymeric calcium phosphate cements modifies their setting behavior. This modification can enhance the mechanical properties of dental materials and bone graft substitutes, improving their performance in biomedical applications .
Fluoride Adsorption
Zirconium(IV)-impregnated materials have shown efficacy in adsorbing fluoride ions from aqueous solutions. This property is particularly relevant for water treatment processes aimed at reducing fluoride concentrations to safe levels .
Environmental Applications
Fluoride Removal Technologies
The compound has been investigated for its potential in environmental remediation, specifically for the removal of fluoride from contaminated water sources. Studies demonstrate that zirconium(IV) fluoride can be effective in adsorbing fluoride ions, thereby reducing toxicity levels in water supplies .
Case Studies
Mécanisme D'action
The mechanism of action of zirconium(IV) fluoride hydrate depends on its specific application. In dental materials, for example, it acts by releasing fluoride ions, which help in the remineralization of tooth enamel and prevention of dental caries. In optical fibers, its low refractive index and high transparency contribute to efficient light transmission.
Comparaison Avec Des Composés Similaires
Zirconium(IV) Chloride (ZrCl₄)
- Molecular Formula : ZrCl₄ .
- Molecular Weight : 233.03 g/mol .
- Properties : Anhydrous, hygroscopic, and highly reactive. Unlike ZrF₄·xH₂O, ZrCl₄ lacks water ligands and is more volatile.
- Applications : Catalyst in organic synthesis, precursor for zirconium metal production .
- Solubility: Soluble in polar solvents like ethanol and water, whereas ZrF₄·xH₂O has variable solubility depending on hydration state .
Zirconyl Nitrate Hydrate (ZrO(NO₃)₂·xH₂O)
- Molecular Formula : H₂N₂O₈Zr .
- Molecular Weight : ~231.23–249.25 g/mol .
- Properties : Water-soluble, acidic, and thermally unstable compared to ZrF₄·xH₂O .
- Applications : Catalyst precursor, ceramic material synthesis, and nuclear industry .
- Safety : Corrosive (R34) and reacts with reducing agents .
Zirconyl Chloride Hydrate (ZrOCl₂·8H₂O)
- Molecular Formula : ZrOCl₂·8H₂O .
- Molecular Weight : 322.25 g/mol .
- Properties : Highly water-soluble and less thermally stable than ZrF₄·xH₂O .
- Applications : Antiperspirant production, tanning agents, and zirconium oxide precursors .
Data Table: Comparative Analysis
Research Findings and Key Differences
- Fluoride Adsorption Efficiency : ZrF₄·xH₂O outperforms zirconium-doped polypyrrole composites in fluoride removal due to its higher Zr(IV) content and structural stability .
- Thermal Stability : ZrF₄·xH₂O is more resistant to hydrolysis than zirconyl nitrate or chloride hydrates, which decompose at lower temperatures .
- Synthetic Utility : Unlike ZrCl₄, ZrF₄·xH₂O is less prone to forming volatile byproducts, making it safer for controlled synthesis of fluoride complexes .
Activité Biologique
Zirconium(IV) fluoride hydrate (ZrF₄·xH₂O) is a compound that has garnered attention due to its unique properties and potential applications in various fields, particularly in environmental science and medicine. This article explores the biological activity of this compound, focusing on its mechanisms of action, adsorption capabilities, and potential implications for health and safety.
This compound is characterized by its coordination chemistry, where zirconium exists in a +4 oxidation state. The compound typically forms complexes with fluoride ions, which are crucial for its biological interactions. The structure of ZrF₄·xH₂O allows it to engage in Lewis acid-base interactions, making it effective in adsorbing fluoride ions from aqueous solutions.
Fluoride Adsorption
One of the primary biological activities of this compound is its ability to adsorb fluoride ions from contaminated water sources. Studies have shown that zirconium-based materials exhibit significant fluoride removal capacities, making them suitable for environmental remediation. For instance:
- Adsorption Capacity : Research indicates that zirconium(IV)-impregnated materials can achieve fluoride adsorption capacities ranging from 2.29 to 17.70 mg/g depending on the conditions such as pH and concentration of fluoride ions in solution .
- Optimal Conditions : The maximum adsorption capacity is often observed at slightly acidic to neutral pH levels (around pH 6.5 to 7), where electrostatic interactions between Zr(IV) and fluoride ions are maximized .
The mechanisms through which this compound functions include:
- Ion Exchange : The interaction between zirconium ions and fluoride ions involves ion exchange processes, where hydroxyl groups on the zirconium surface are replaced by fluoride ions .
- Lewis Acid-Base Interactions : Zirconium acts as a Lewis acid, facilitating strong interactions with fluoride ions, which enhances its adsorption capacity .
Case Studies
Several studies have illustrated the effectiveness of Zirconium(IV) compounds in removing fluoride from water:
- Zirconium(IV)-Doped Chitosan : A study demonstrated that chitosan doped with 20% zirconium(IV) exhibited excellent defluoridation capabilities with an optimal sorbent dose of 10 g/L under neutral pH conditions . The maximum defluoridation rate reached up to 90% at certain concentrations.
- Amino Acid Complexes : Research involving amino acid complexes of zirconium showed enhanced fluoride adsorption due to the presence of carboxylate groups, which improved ion-exchange interactions with fluoride anions . This approach yielded a higher adsorption capacity compared to traditional methods.
Health Implications
While this compound has beneficial applications in water purification, it also poses health risks if not handled properly:
- Toxicity : The compound is corrosive and can cause severe injuries upon contact with skin or eyes, and inhalation may lead to respiratory issues such as chemical pneumonitis .
- Environmental Concerns : Continuous exposure to high levels of fluoride can lead to skeletal and dental fluorosis, necessitating effective removal strategies from drinking water sources .
Q & A
Q. Basic: What methodologies are recommended for synthesizing zirconium(IV) fluoride hydrate complexes for dental resin applications?
Answer: A common synthesis involves dissolving this compound in methanol with aqueous HF, followed by reaction with functional monomers (e.g., methacrylate derivatives). Post-reaction, solvent evaporation under controlled conditions (e.g., over Ca(OH)₂ to neutralize HF) yields fluoride-releasing complexes. Structural validation via FT-IR (to confirm Zr–F bonds), NMR (to track ligand coordination), and ESMS (for molecular weight confirmation) is critical .
Q. Basic: Which analytical techniques are essential for confirming the structural integrity of this compound complexes?
Answer: Key techniques include:
- FT-IR : Identifies Zr–F vibrational modes (~500–600 cm⁻¹) and ligand bonding.
- NMR : Monitors shifts in ligand protons or carbons upon Zr coordination.
- XRD : Detects crystallinity and phase composition, especially after thermal treatments (e.g., tetragonal vs. monoclinic ZrO₂ phases post-annealing) .
- SEM-EDS : For morphological and elemental analysis in composite materials .
Q. Advanced: How does this compound interact with biomolecules like L-histidine, and what experimental challenges arise?
Answer: Mixing ZrO(NO₃)₂·xH₂O with L-histidine in aqueous solutions can form hydrogels due to Zr⁴⁺ coordination with histidine’s imidazole group. Centrifugation separates the amorphous zirconium hydroxide phase, while residual solutes require slow evaporation for crystallization. Challenges include amorphous product formation and difficulty in indexing XRD patterns, necessitating complementary techniques like thermal gravimetric analysis (TGA) to study decomposition pathways .
Q. Advanced: What contradictions exist in fluoride adsorption studies using zirconium-based adsorbents, and how can they be resolved?
Answer: Discrepancies in adsorption capacity (e.g., 5–40 mg F⁻/g) arise from variations in:
- pH : Optimal adsorption occurs at pH 3–5 due to Zr–F electrostatic interactions.
- Co-existing ions : PO₄³⁻ and SO₄²⁻ compete with F⁻ for binding sites.
- Material porosity : Nanostructured Zr(OH)₄ exhibits higher surface area than bulk hydrates. Resolving contradictions requires standardized testing (fixed pH, ionic strength) and comparative studies using control adsorbents .
Q. Methodological: How can fluoride ion release from this compound be quantified accurately?
Answer: Use a fluoride ion-selective electrode (ISE) calibrated with NaF standards (0.1–10 ppm F⁻). Key steps:
- Prepare samples in plasticware to avoid glass-derived fluoride contamination.
- Add Total Ionic Strength Adjustment Buffer (TISAB) to mitigate matrix effects.
- Validate measurements against ICP-MS for trace-level accuracy .
Q. Advanced: How should researchers design experiments to analyze thermal decomposition products of this compound complexes?
Answer: Conduct controlled heating (e.g., 100–600°C) in a furnace under inert atmosphere, followed by:
- TGA-DSC : Track mass loss and phase transitions.
- XRD : Identify crystalline phases (e.g., ZrO₂ polymorphs).
- FT-IR : Monitor loss of hydrate and fluoride ligands. Note that residual carbon from organic ligands may require calcination at higher temperatures .
Q. Basic: What safety protocols are critical when handling this compound?
Answer:
- Use PPE: Nitrile gloves, lab coat, and safety goggles to prevent skin/eye contact.
- Work in a fume hood to avoid HF vapor exposure.
- Neutralize waste with Ca(OH)₂ before disposal.
- Store in sealed containers under dry, inert conditions .
Q. Advanced: How can zirconium(IV) hydroxide-incorporated nanofibers be optimized for chemical warfare agent (CWA) degradation?
Answer:
- Polymer selection : Use polyvinyl butyral (PVB) for enhanced solvent resistance.
- Nanoparticle loading : Increase Zr(OH)₄ content to 10–15 wt% to boost active sites.
- Morphology control : Electrospinning parameters (voltage, flow rate) tune fiber diameter (100–500 nm) for higher surface area.
- Performance testing : Evaluate CWA degradation in non-polar solvents using GC-MS and compare with control materials .
Q. Basic: What solvents are suitable for dissolving this compound, and how does solubility impact experimental design?
Answer: Methanol and aqueous HF are effective solvents due to Zr–F stability. Avoid water-rich systems unless ligands stabilize Zr⁴⁺ against hydrolysis. Solubility limitations in polar aprotic solvents (e.g., DMF) may require sonication or elevated temperatures .
Q. Advanced: How can researchers resolve discrepancies in identifying zirconium-fluoride precipitates formed during hydrolysis?
Answer: Precipitates often contain mixed phases (e.g., hydrous ZrO₂, ZrF₄·xH₂O). Use:
- XPS : Quantify F/Zr atomic ratios.
- Synchrotron XRD : Detect low-crystallinity phases.
- Chemical digestion : Measure soluble F⁻ and Zr⁴⁺ post-precipitation to infer stoichiometry .
Propriétés
IUPAC Name |
tetrafluorozirconium;hydrate | |
---|---|---|
Source | PubChem | |
URL | https://pubchem.ncbi.nlm.nih.gov | |
Description | Data deposited in or computed by PubChem | |
InChI |
InChI=1S/4FH.H2O.Zr/h4*1H;1H2;/q;;;;;+4/p-4 | |
Source | PubChem | |
URL | https://pubchem.ncbi.nlm.nih.gov | |
Description | Data deposited in or computed by PubChem | |
InChI Key |
GORLMDNEWNWWBP-UHFFFAOYSA-J | |
Source | PubChem | |
URL | https://pubchem.ncbi.nlm.nih.gov | |
Description | Data deposited in or computed by PubChem | |
Canonical SMILES |
O.F[Zr](F)(F)F | |
Source | PubChem | |
URL | https://pubchem.ncbi.nlm.nih.gov | |
Description | Data deposited in or computed by PubChem | |
Molecular Formula |
F4H2OZr | |
Source | PubChem | |
URL | https://pubchem.ncbi.nlm.nih.gov | |
Description | Data deposited in or computed by PubChem | |
Molecular Weight |
185.23 g/mol | |
Source | PubChem | |
URL | https://pubchem.ncbi.nlm.nih.gov | |
Description | Data deposited in or computed by PubChem | |
CAS No. |
15298-38-1 | |
Record name | Zirconium(IV) fluoride hydrate | |
Source | European Chemicals Agency (ECHA) | |
URL | https://echa.europa.eu/information-on-chemicals | |
Description | The European Chemicals Agency (ECHA) is an agency of the European Union which is the driving force among regulatory authorities in implementing the EU's groundbreaking chemicals legislation for the benefit of human health and the environment as well as for innovation and competitiveness. | |
Explanation | Use of the information, documents and data from the ECHA website is subject to the terms and conditions of this Legal Notice, and subject to other binding limitations provided for under applicable law, the information, documents and data made available on the ECHA website may be reproduced, distributed and/or used, totally or in part, for non-commercial purposes provided that ECHA is acknowledged as the source: "Source: European Chemicals Agency, http://echa.europa.eu/". Such acknowledgement must be included in each copy of the material. ECHA permits and encourages organisations and individuals to create links to the ECHA website under the following cumulative conditions: Links can only be made to webpages that provide a link to the Legal Notice page. | |
Avertissement et informations sur les produits de recherche in vitro
Veuillez noter que tous les articles et informations sur les produits présentés sur BenchChem sont destinés uniquement à des fins informatives. Les produits disponibles à l'achat sur BenchChem sont spécifiquement conçus pour des études in vitro, qui sont réalisées en dehors des organismes vivants. Les études in vitro, dérivées du terme latin "in verre", impliquent des expériences réalisées dans des environnements de laboratoire contrôlés à l'aide de cellules ou de tissus. Il est important de noter que ces produits ne sont pas classés comme médicaments et n'ont pas reçu l'approbation de la FDA pour la prévention, le traitement ou la guérison de toute condition médicale, affection ou maladie. Nous devons souligner que toute forme d'introduction corporelle de ces produits chez les humains ou les animaux est strictement interdite par la loi. Il est essentiel de respecter ces directives pour assurer la conformité aux normes légales et éthiques en matière de recherche et d'expérimentation.