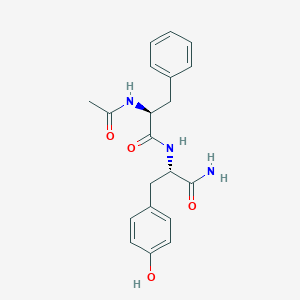
Ac-Phe-Tyr-NH2
Vue d'ensemble
Description
Acetyl-phenylalanine-tyrosine-amide (Ac-Phe-Tyr-NH2) is a synthetic dipeptide composed of acetylated phenylalanine and tyrosine residues. This compound is of interest due to its potential biological activities and applications in various fields, including medicinal chemistry and peptide-based drug development.
Mécanisme D'action
Target of Action
The primary target of Ac-Phe-Tyr-NH2 is the μ-opioid receptor . This receptor is a G protein-coupled receptor that plays a crucial role in mediating the effects of opioids. It is involved in pain regulation, mood control, immune response, and other physiological functions.
Mode of Action
This compound interacts with its target, the μ-opioid receptor, through a mechanism that is presumed to be analogous to that of endogenous peptides . This interaction leads to the desensitization of the receptor, which can result in analgesic effects .
Biochemical Pathways
This results in hyperpolarization of the cell membrane and inhibition of neurotransmitter release .
Pharmacokinetics
It is known that the compound can be administered subcutaneously . The replacement of D-Ala2 by D-Arg2 in the starting amino-acid sequence of dermorphine, from which this compound is derived, makes the molecule more resistant to enzymatic degradation .
Result of Action
The primary result of this compound’s action is its analgesic effect. In animal models, it has been shown to significantly prolong tail-flick latency when administered in the dose range of 0.01 – 5 mg/kg . The analgesic effect of a single subcutaneous injection of this compound at 1 mg/kg exceeds the effect of morphine hydrochloride at the same dose .
Action Environment
The action of this compound can be influenced by various environmental factors. For instance, the solvent used in the synthesis of this compound can affect its stability and efficacy
Analyse Biochimique
Biochemical Properties
Ac-Phe-Tyr-NH2 plays a significant role in biochemical reactions. It interacts with the enzyme α-chymotrypsin in the synthesis of N-Ac-Phe-Tyr-NH2 . This interaction involves a condensation reaction of N-acetyl phenylalanine ethyl ester (N-Ac-Phe-OEt) and tyrosinamide (Tyr-NH2) .
Cellular Effects
It is known that the compound influences cell function through its interactions with various enzymes and proteins .
Molecular Mechanism
The molecular mechanism of this compound involves its interaction with α-chymotrypsin. This interaction leads to the synthesis of N-Ac-Phe-Tyr-NH2, which has angiotensin I-converting enzyme (ACE) inhibitor activity .
Metabolic Pathways
This compound is involved in several metabolic pathways. It interacts with enzymes and cofactors, potentially affecting metabolic flux or metabolite levels .
Méthodes De Préparation
Synthetic Routes and Reaction Conditions
Acetyl-phenylalanine-tyrosine-amide can be synthesized through an enzymatic condensation reaction. One common method involves the use of α-chymotrypsin as a catalyst. The reaction typically involves the condensation of N-acetyl phenylalanine ethyl ester (N-Ac-Phe-OEt) and tyrosinamide (Tyr-NH2) in the presence of a suitable solvent mixture. The optimal solvent mixture for this reaction has been found to be a combination of Tris-HCl buffer, dimethylsulfoxide (DMSO), and acetonitrile in a ratio of 2:1:1 . The reaction conditions include a reaction time of approximately 7.4 minutes, a temperature of 28.1°C, and an enzyme activity of 98.9 U .
Industrial Production Methods
While specific industrial production methods for Acetyl-phenylalanine-tyrosine-amide are not widely documented, the enzymatic synthesis approach described above can be scaled up for industrial applications. The use of response surface methodology (RSM) and central composite rotatable design (CCRD) can help optimize the reaction parameters to achieve high yields and efficiency .
Analyse Des Réactions Chimiques
Types of Reactions
Acetyl-phenylalanine-tyrosine-amide can undergo various chemical reactions, including:
Oxidation: The phenol group in the tyrosine residue can be oxidized to form quinones.
Reduction: The amide bond can be reduced under specific conditions.
Substitution: The phenol group can undergo electrophilic aromatic substitution reactions.
Common Reagents and Conditions
Reduction: Reducing agents like lithium aluminum hydride (LiAlH4) can be employed for the reduction of the amide bond.
Substitution: Electrophilic aromatic substitution can be carried out using reagents like bromine or nitrating agents under acidic conditions.
Major Products Formed
Oxidation: Quinone derivatives.
Reduction: Reduced amide derivatives.
Substitution: Halogenated or nitrated phenylalanine-tyrosine-amide derivatives.
Applications De Recherche Scientifique
Acetyl-phenylalanine-tyrosine-amide has several scientific research applications, including:
Chemistry: Used as a model compound for studying peptide synthesis and modification techniques.
Biology: Investigated for its potential role in enzyme inhibition and protein-protein interactions.
Industry: Utilized in the development of peptide-based materials and hydrogels for various applications.
Comparaison Avec Des Composés Similaires
Similar Compounds
Acetyl-phenylalanine-tyrosine: Similar structure but lacks the amide group.
Phenylalanine-tyrosine-amide: Lacks the acetyl group.
Acetyl-tyrosine-phenylalanine-amide: Different sequence of amino acids.
Uniqueness
Acetyl-phenylalanine-tyrosine-amide is unique due to its specific sequence and modifications, which confer distinct biological activities and chemical properties. The acetylation and amidation enhance its stability and potential interactions with biological targets, making it a valuable compound for research and therapeutic applications.
Activité Biologique
Acetyl-phenylalanine-tyrosine-amide (Ac-Phe-Tyr-NH2) is a synthetic dipeptide that has garnered attention due to its potential biological activities. This article explores its biological activity, mechanisms of action, pharmacological effects, and relevant case studies.
Overview of this compound
This compound is composed of acetylated phenylalanine and tyrosine residues. Its synthesis typically involves enzymatic methods, particularly using α-chymotrypsin, which catalyzes the condensation of N-acetyl phenylalanine and tyrosinamide. This compound is primarily studied for its interactions with the μ-opioid receptor and its role as an angiotensin I-converting enzyme (ACE) inhibitor, making it relevant in pain management and hypertension treatment.
Interaction with μ-Opioid Receptor
This compound exhibits analgesic properties by interacting with the μ-opioid receptor. This interaction leads to hyperpolarization of the cell membrane and inhibition of neurotransmitter release, resulting in pain relief. In animal models, administration of this compound has shown a significant increase in tail-flick latency, indicating enhanced analgesic effects at doses ranging from 0.01 to 5 mg/kg .
ACE Inhibition
The compound acts as a competitive inhibitor of ACE, preventing the conversion of Angiotensin I to Angiotensin II. This inhibition promotes vasodilation and reduces blood pressure, highlighting its potential as an antihypertensive agent .
Pharmacokinetics
This compound can be administered subcutaneously, where it demonstrates stability and efficacy. Its pharmacokinetic profile suggests that it may be suitable for therapeutic applications requiring sustained release and activity .
This compound influences various biochemical pathways:
- Enzyme Interaction : The compound interacts with α-chymotrypsin during synthesis, impacting the efficiency of peptide bond formation .
- Cellular Effects : It modulates cellular functions through interactions with enzymes and proteins involved in metabolic pathways .
Synthesis Optimization
A study optimized the synthesis of this compound using a mixture of solvents (Tris-HCl buffer, DMSO, acetonitrile) to enhance yield. The optimal conditions included a reaction time of 7.4 minutes at 28.1°C, resulting in a yield of approximately 85.5% .
Pharmacological Activity
Research indicates that this compound exhibits significant pharmacological activity. It was found to stabilize against degradation compared to other peptides like H-Tyr-Phe-OH, which was metabolized rapidly . This stability enhances its potential for therapeutic applications.
Data Table: Summary of Biological Activities
Activity | Mechanism | Outcome |
---|---|---|
Analgesic Effect | μ-opioid receptor interaction | Increased tail-flick latency |
Antihypertensive Activity | ACE inhibition | Reduced blood pressure |
Enzyme Interaction | α-chymotrypsin catalysis | Enhanced peptide synthesis yield |
Stability in Biological Systems | Resistance to metabolic degradation | Sustained biological activity |
Future Directions
Research on this compound could focus on:
- Further Mechanistic Studies : Understanding the precise binding interactions with ACE and μ-opioid receptors.
- Clinical Trials : Evaluating its efficacy in human subjects for pain management and hypertension.
- Synthetic Methodologies : Exploring alternative synthesis routes that may improve yield or reduce costs.
Propriétés
IUPAC Name |
(2S)-2-acetamido-N-[(2S)-1-amino-3-(4-hydroxyphenyl)-1-oxopropan-2-yl]-3-phenylpropanamide | |
---|---|---|
Source | PubChem | |
URL | https://pubchem.ncbi.nlm.nih.gov | |
Description | Data deposited in or computed by PubChem | |
InChI |
InChI=1S/C20H23N3O4/c1-13(24)22-18(12-14-5-3-2-4-6-14)20(27)23-17(19(21)26)11-15-7-9-16(25)10-8-15/h2-10,17-18,25H,11-12H2,1H3,(H2,21,26)(H,22,24)(H,23,27)/t17-,18-/m0/s1 | |
Source | PubChem | |
URL | https://pubchem.ncbi.nlm.nih.gov | |
Description | Data deposited in or computed by PubChem | |
InChI Key |
NRNUHNDVXOFSJO-ROUUACIJSA-N | |
Source | PubChem | |
URL | https://pubchem.ncbi.nlm.nih.gov | |
Description | Data deposited in or computed by PubChem | |
Canonical SMILES |
CC(=O)NC(CC1=CC=CC=C1)C(=O)NC(CC2=CC=C(C=C2)O)C(=O)N | |
Source | PubChem | |
URL | https://pubchem.ncbi.nlm.nih.gov | |
Description | Data deposited in or computed by PubChem | |
Isomeric SMILES |
CC(=O)N[C@@H](CC1=CC=CC=C1)C(=O)N[C@@H](CC2=CC=C(C=C2)O)C(=O)N | |
Source | PubChem | |
URL | https://pubchem.ncbi.nlm.nih.gov | |
Description | Data deposited in or computed by PubChem | |
Molecular Formula |
C20H23N3O4 | |
Source | PubChem | |
URL | https://pubchem.ncbi.nlm.nih.gov | |
Description | Data deposited in or computed by PubChem | |
DSSTOX Substance ID |
DTXSID10427269 | |
Record name | Ac-Phe-Tyr-NH2 | |
Source | EPA DSSTox | |
URL | https://comptox.epa.gov/dashboard/DTXSID10427269 | |
Description | DSSTox provides a high quality public chemistry resource for supporting improved predictive toxicology. | |
Molecular Weight |
369.4 g/mol | |
Source | PubChem | |
URL | https://pubchem.ncbi.nlm.nih.gov | |
Description | Data deposited in or computed by PubChem | |
CAS No. |
19361-52-5 | |
Record name | Ac-Phe-Tyr-NH2 | |
Source | EPA DSSTox | |
URL | https://comptox.epa.gov/dashboard/DTXSID10427269 | |
Description | DSSTox provides a high quality public chemistry resource for supporting improved predictive toxicology. | |
Record name | N-ACETYL-L-PHENYLALANYL-L-TYROSINAMIDE | |
Source | European Chemicals Agency (ECHA) | |
URL | https://echa.europa.eu/information-on-chemicals | |
Description | The European Chemicals Agency (ECHA) is an agency of the European Union which is the driving force among regulatory authorities in implementing the EU's groundbreaking chemicals legislation for the benefit of human health and the environment as well as for innovation and competitiveness. | |
Explanation | Use of the information, documents and data from the ECHA website is subject to the terms and conditions of this Legal Notice, and subject to other binding limitations provided for under applicable law, the information, documents and data made available on the ECHA website may be reproduced, distributed and/or used, totally or in part, for non-commercial purposes provided that ECHA is acknowledged as the source: "Source: European Chemicals Agency, http://echa.europa.eu/". Such acknowledgement must be included in each copy of the material. ECHA permits and encourages organisations and individuals to create links to the ECHA website under the following cumulative conditions: Links can only be made to webpages that provide a link to the Legal Notice page. | |
Retrosynthesis Analysis
AI-Powered Synthesis Planning: Our tool employs the Template_relevance Pistachio, Template_relevance Bkms_metabolic, Template_relevance Pistachio_ringbreaker, Template_relevance Reaxys, Template_relevance Reaxys_biocatalysis model, leveraging a vast database of chemical reactions to predict feasible synthetic routes.
One-Step Synthesis Focus: Specifically designed for one-step synthesis, it provides concise and direct routes for your target compounds, streamlining the synthesis process.
Accurate Predictions: Utilizing the extensive PISTACHIO, BKMS_METABOLIC, PISTACHIO_RINGBREAKER, REAXYS, REAXYS_BIOCATALYSIS database, our tool offers high-accuracy predictions, reflecting the latest in chemical research and data.
Strategy Settings
Precursor scoring | Relevance Heuristic |
---|---|
Min. plausibility | 0.01 |
Model | Template_relevance |
Template Set | Pistachio/Bkms_metabolic/Pistachio_ringbreaker/Reaxys/Reaxys_biocatalysis |
Top-N result to add to graph | 6 |
Feasible Synthetic Routes
Q1: How does Ac-Phe-Tyr-NH2, as a potential antihypertensive agent, interact with its target and what are the downstream effects?
A: this compound (N-Acetyl-Phenylalanine-Tyrosine-Amide) acts as a competitive inhibitor of Angiotensin I-Converting Enzyme (ACE). [, , ] While its exact binding mechanism is still under investigation, it's believed to interact with the active site of ACE, preventing the conversion of Angiotensin I to Angiotensin II. [] This inhibition of Angiotensin II production leads to vasodilation and a subsequent decrease in blood pressure. []
Q2: What are the structural characteristics of this compound?
A: this compound is a modified dipeptide composed of N-acetyl-Phenylalanine and Tyrosine-amide. While specific spectroscopic data isn't detailed within the provided abstracts, its molecular formula can be deduced as C20H23N3O4 with a molecular weight of approximately 369.4 g/mol. []
Q3: What enzymatic approaches have been explored for the synthesis of this compound?
A: Researchers have investigated both α-chymotrypsin and porcine pancreatic lipase (PPL) for the enzymatic synthesis of this compound. [, ] These studies focused on optimizing reaction conditions like solvent composition, temperature, enzyme concentration, and substrate ratios to maximize yield. Interestingly, PPL exhibited comparable catalytic efficiency to α-chymotrypsin in synthesizing this specific peptide. []
Q4: How does modifying the structure of this compound impact its interaction with the PepT1 transporter?
A: Research suggests that the N-terminus of this compound plays a crucial role in binding to the intestinal peptide transporter PepT1. [] Blocking the N-terminus with an acetyl group significantly reduces the binding affinity. For example, Ac-Phe-Tyr shows significantly weaker interaction with PepT1 compared to the unblocked Phe-Tyr. [] Additionally, blocking the C-terminus with an amide group has a less pronounced effect on binding affinity compared to N-terminal modifications. []
Q5: What are the current limitations in the research on this compound and what future directions could be explored?
A: The provided abstracts primarily focus on the synthesis and in vitro characterization of this compound. Further research is needed to explore its in vivo efficacy, pharmacokinetics, and potential toxicity. [, , ] Investigating the long-term effects, optimal dosage, and potential side effects in animal models and eventually clinical trials would be crucial for translating this compound into a viable antihypertensive therapeutic. Further exploration of the structure-activity relationship could lead to the development of derivatives with improved potency and selectivity for ACE.
Avertissement et informations sur les produits de recherche in vitro
Veuillez noter que tous les articles et informations sur les produits présentés sur BenchChem sont destinés uniquement à des fins informatives. Les produits disponibles à l'achat sur BenchChem sont spécifiquement conçus pour des études in vitro, qui sont réalisées en dehors des organismes vivants. Les études in vitro, dérivées du terme latin "in verre", impliquent des expériences réalisées dans des environnements de laboratoire contrôlés à l'aide de cellules ou de tissus. Il est important de noter que ces produits ne sont pas classés comme médicaments et n'ont pas reçu l'approbation de la FDA pour la prévention, le traitement ou la guérison de toute condition médicale, affection ou maladie. Nous devons souligner que toute forme d'introduction corporelle de ces produits chez les humains ou les animaux est strictement interdite par la loi. Il est essentiel de respecter ces directives pour assurer la conformité aux normes légales et éthiques en matière de recherche et d'expérimentation.