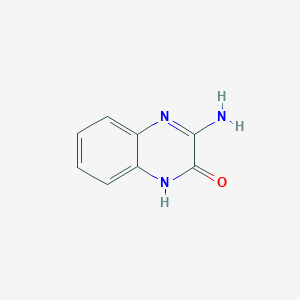
3-Aminoquinoxalin-2(1H)-one
Vue d'ensemble
Description
3-Aminoquinoxalin-2(1H)-one is a heterocyclic compound that belongs to the quinoxaline family This compound is characterized by a quinoxaline ring system with an amino group at the third position and a keto group at the second position
Applications De Recherche Scientifique
3-Aminoquinoxalin-2(1H)-one has a wide range of applications in scientific research:
Chemistry: It is used as a building block for the synthesis of more complex heterocyclic compounds.
Biology: The compound exhibits antimicrobial, antiviral, and anticancer activities, making it a valuable tool in biological research.
Medicine: Due to its biological activities, this compound is explored for potential therapeutic applications, including drug development.
Industry: The compound is used in the development of agrochemicals and other industrial products
Mécanisme D'action
Target of Action
The primary target of 3-Aminoquinoxalin-2(1H)-one is the C-3 position of the quinoxalin-2(1H)-one molecule . This target plays a crucial role in the compound’s interaction with other molecules, particularly in reactions involving amination .
Mode of Action
This compound interacts with its targets through a process known as oxidative amination . This involves the addition of an amine group to the C-3 position of the quinoxalin-2(1H)-one molecule . The reaction is facilitated by copper catalysis .
Biochemical Pathways
The primary biochemical pathway affected by this compound is the C–H functionalization pathway . This pathway involves the formation of new bonds (C–C, C–N, C–P, C–S, and C–O) via the functionalization of the C–H bond of the quinoxalin-2(1H)-one molecule . The downstream effects of this pathway include the creation of a wide variety of functionalized quinoxalin-2(1H)-one derivatives .
Pharmacokinetics
The compound’s high yield in reactions suggests that it may have good bioavailability.
Result of Action
The result of this compound’s action is the formation of a wide variety of This compound derivatives . These derivatives are formed with good functional group tolerance , indicating that the compound’s action can result in a diverse range of molecular structures.
Action Environment
The action of this compound is influenced by various environmental factors. For instance, the copper-catalysed oxidative amination reaction is performed under mild reaction conditions , suggesting that the compound’s action, efficacy, and stability may be sensitive to temperature and other environmental conditions.
Orientations Futures
Future developments of reactions involving 3-Aminoquinoxalin-2(1H)-one are envisaged, such as the simultaneous construction of C–C and C–B/SI/P/F/I/SE bonds through multi-component reactions; the construction of fused ring and macrocyclic compounds; asymmetric synthesis; green chemistry; bionic structures and other fields .
Analyse Biochimique
Biochemical Properties
The role of 3-Aminoquinoxalin-2(1H)-one in biochemical reactions primarily involves the process of amination . It interacts with primary or secondary amines as the nitrogen sources in the presence of a copper catalyst . The nature of these interactions involves the formation of a wide variety of 3-aminoquinoxalin-2(1H)-ones .
Cellular Effects
Quinoxalin-2(1H)-ones, the broader class of compounds to which this compound belongs, are known to exhibit significant bioactive properties, including antiviral, antidiabetic, antimicrobial, antibacterial, anticancer, anti-inflammatory, and protein kinase inhibitory activities .
Molecular Mechanism
The molecular mechanism of this compound involves copper-catalyzed oxidative C-3 amination . This process allows for the formation of a wide variety of 3-aminoquinoxalin-2(1H)-ones
Temporal Effects in Laboratory Settings
The synthesis of this compound has been demonstrated to be efficient, with good functional group tolerance .
Metabolic Pathways
The compound is involved in the process of amination, which is a key part of many metabolic pathways .
Méthodes De Préparation
Synthetic Routes and Reaction Conditions: The synthesis of 3-Aminoquinoxalin-2(1H)-one typically involves the functionalization of quinoxalin-2(1H)-one. One common method is the copper-catalyzed oxidative amination of quinoxalin-2(1H)-ones with primary or secondary amines. This method provides high yields and good functional group tolerance . Another approach involves the transition metal-free C-3 functionalization of quinoxalin-2(1H)-ones, which is considered an environmentally benign synthetic route .
Industrial Production Methods: Industrial production methods for this compound often rely on scalable and efficient synthetic strategies. The copper-catalyzed oxidative amination method is favored due to its high yield and mild reaction conditions, making it suitable for large-scale production .
Analyse Des Réactions Chimiques
Types of Reactions: 3-Aminoquinoxalin-2(1H)-one undergoes various types of chemical reactions, including:
Oxidation: The compound can be oxidized to form quinoxaline derivatives.
Reduction: Reduction reactions can convert the keto group to a hydroxyl group.
Substitution: The amino group at the third position can participate in substitution reactions, leading to the formation of various derivatives.
Common Reagents and Conditions:
Oxidation: Common oxidizing agents include potassium permanganate and hydrogen peroxide.
Reduction: Reducing agents such as sodium borohydride and lithium aluminum hydride are used.
Substitution: Substitution reactions often involve reagents like alkyl halides and acyl chlorides under basic or acidic conditions.
Major Products: The major products formed from these reactions include various substituted quinoxaline derivatives, which can exhibit different biological activities and properties .
Comparaison Avec Des Composés Similaires
Quinoxalin-2(1H)-one: The parent compound without the amino group.
3-Methylquinoxalin-2(1H)-one: A similar compound with a methyl group instead of an amino group at the third position.
3-Phenylquinoxalin-2(1H)-one: A compound with a phenyl group at the third position.
Uniqueness: 3-Aminoquinoxalin-2(1H)-one is unique due to the presence of the amino group at the third position, which imparts distinct chemical and biological properties. This functional group allows for a wide range of chemical modifications and enhances the compound’s biological activities compared to its analogs .
Propriétés
IUPAC Name |
3-amino-1H-quinoxalin-2-one | |
---|---|---|
Source | PubChem | |
URL | https://pubchem.ncbi.nlm.nih.gov | |
Description | Data deposited in or computed by PubChem | |
InChI |
InChI=1S/C8H7N3O/c9-7-8(12)11-6-4-2-1-3-5(6)10-7/h1-4H,(H2,9,10)(H,11,12) | |
Source | PubChem | |
URL | https://pubchem.ncbi.nlm.nih.gov | |
Description | Data deposited in or computed by PubChem | |
InChI Key |
QBBADXPVZMYLKN-UHFFFAOYSA-N | |
Source | PubChem | |
URL | https://pubchem.ncbi.nlm.nih.gov | |
Description | Data deposited in or computed by PubChem | |
Canonical SMILES |
C1=CC=C2C(=C1)NC(=O)C(=N2)N | |
Source | PubChem | |
URL | https://pubchem.ncbi.nlm.nih.gov | |
Description | Data deposited in or computed by PubChem | |
Molecular Formula |
C8H7N3O | |
Source | PubChem | |
URL | https://pubchem.ncbi.nlm.nih.gov | |
Description | Data deposited in or computed by PubChem | |
DSSTOX Substance ID |
DTXSID70406176 | |
Record name | 3-amino-1H-quinoxalin-2-one | |
Source | EPA DSSTox | |
URL | https://comptox.epa.gov/dashboard/DTXSID70406176 | |
Description | DSSTox provides a high quality public chemistry resource for supporting improved predictive toxicology. | |
Molecular Weight |
161.16 g/mol | |
Source | PubChem | |
URL | https://pubchem.ncbi.nlm.nih.gov | |
Description | Data deposited in or computed by PubChem | |
CAS No. |
35015-91-9 | |
Record name | 3-amino-1H-quinoxalin-2-one | |
Source | EPA DSSTox | |
URL | https://comptox.epa.gov/dashboard/DTXSID70406176 | |
Description | DSSTox provides a high quality public chemistry resource for supporting improved predictive toxicology. | |
Synthesis routes and methods
Procedure details
Retrosynthesis Analysis
AI-Powered Synthesis Planning: Our tool employs the Template_relevance Pistachio, Template_relevance Bkms_metabolic, Template_relevance Pistachio_ringbreaker, Template_relevance Reaxys, Template_relevance Reaxys_biocatalysis model, leveraging a vast database of chemical reactions to predict feasible synthetic routes.
One-Step Synthesis Focus: Specifically designed for one-step synthesis, it provides concise and direct routes for your target compounds, streamlining the synthesis process.
Accurate Predictions: Utilizing the extensive PISTACHIO, BKMS_METABOLIC, PISTACHIO_RINGBREAKER, REAXYS, REAXYS_BIOCATALYSIS database, our tool offers high-accuracy predictions, reflecting the latest in chemical research and data.
Strategy Settings
Precursor scoring | Relevance Heuristic |
---|---|
Min. plausibility | 0.01 |
Model | Template_relevance |
Template Set | Pistachio/Bkms_metabolic/Pistachio_ringbreaker/Reaxys/Reaxys_biocatalysis |
Top-N result to add to graph | 6 |
Feasible Synthetic Routes
Q1: What are the key challenges in synthesizing unsymmetrically substituted 3-aminoquinoxalin-2(1H)-ones?
A: Synthesizing unsymmetrically substituted 3-aminoquinoxalin-2(1H)-ones often leads to the formation of regioisomers, posing a significant challenge. The specific isomer formed depends on the reactivity of the ring-closure reagent used in the synthesis. [] For example, using reagents like oxalomonoimidic acid esters or chloro(methylimino)acetic acid ethyl ester can lead to different ratios of isomers. []
Q2: How can NMR spectroscopy be used to distinguish between isomers of 3-aminoquinoxalin-2(1H)-one?
A: NMR techniques, specifically ¹H, ¹³C, and ¹⁵N NMR, are invaluable for identifying and characterizing the different isomers of this compound. [, ] These techniques provide detailed information about the arrangement of atoms within the molecule, allowing researchers to differentiate between isomers based on their unique spectral signatures.
Q3: What unexpected regioselectivity is observed during the nitration of 3-aminoquinoxalin-2(1H)-ones?
A: Nitration of 6,7-disubstituted-3-aminoquinoxalin-2(1H)-ones unexpectedly favors substitution at the 8-position, regardless of the electronic or steric effects of substituents on the aromatic ring or the amino group. [] This regioselectivity, confirmed through theoretical calculations and NMR studies, suggests that the nitronium ion preferentially attacks the monoprotonated form of the molecule at the 8-position. []
Q4: Can you describe an efficient method for synthesizing 3-aminoquinoxalin-2(1H)-ones?
A: A highly effective and practical approach involves a copper-catalyzed oxidative amination of quinoxalin-2(1H)-ones using readily available primary or secondary amines. [] This method offers several advantages, including high atom economy, simple operation, mild reaction conditions, and impressive yields of up to 98%. []
Q5: What alternative, metal-free approach exists for synthesizing N-acylated 3-aminoquinoxalin-2(1H)-ones?
A: A recently developed method utilizes visible light and a rhodamine B catalyst to promote the amidation of quinoxalin-2(1H)-ones with amides. [] This approach, conducted under mild, metal-free conditions using air as an oxidant, offers a sustainable and efficient route to diverse N-acylated this compound derivatives. []
Avertissement et informations sur les produits de recherche in vitro
Veuillez noter que tous les articles et informations sur les produits présentés sur BenchChem sont destinés uniquement à des fins informatives. Les produits disponibles à l'achat sur BenchChem sont spécifiquement conçus pour des études in vitro, qui sont réalisées en dehors des organismes vivants. Les études in vitro, dérivées du terme latin "in verre", impliquent des expériences réalisées dans des environnements de laboratoire contrôlés à l'aide de cellules ou de tissus. Il est important de noter que ces produits ne sont pas classés comme médicaments et n'ont pas reçu l'approbation de la FDA pour la prévention, le traitement ou la guérison de toute condition médicale, affection ou maladie. Nous devons souligner que toute forme d'introduction corporelle de ces produits chez les humains ou les animaux est strictement interdite par la loi. Il est essentiel de respecter ces directives pour assurer la conformité aux normes légales et éthiques en matière de recherche et d'expérimentation.