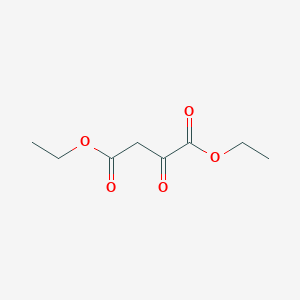
Diethyl oxalacetate
Vue d'ensemble
Description
Diethyl oxalacetate, also known as diethyl oxaloacetate, is an organic compound with the molecular formula C8H12O5. It is a diethyl ester of oxaloacetic acid and is commonly used in organic synthesis as a reagent and intermediate. This compound is known for its role in various chemical reactions and its applications in scientific research and industrial processes.
Mécanisme D'action
Target of Action
Diethyl oxalacetate, also known as diethyl 2-oxobutanedioate, is a chemical compound used in various biochemical reactions. It is often used as a reagent in the synthesis of other compounds, indicating its role in facilitating chemical reactions .
Mode of Action
For instance, it can be used to synthesize spiro[indoline-3,4′-pyrano[2,3-c]pyrazole derivatives via a one-pot reaction with isatins, malononitrile, and hydrazine hydrate . It can also react with various aldehydes and primary amines to produce 2,3-dioxo-4-carboxy-5-substituted pyrrolidines .
Biochemical Pathways
It participates in gluconeogenesis, the urea cycle, the glyoxylate cycle, amino acid synthesis, fatty acid synthesis, and the citric acid cycle .
Result of Action
The molecular and cellular effects of this compound’s action are largely dependent on the specific reactions it is involved in. As a reagent, it contributes to the synthesis of various compounds, which can have diverse effects depending on their structure and function .
Analyse Biochimique
Biochemical Properties
Diethyl oxalacetate is involved in numerous biochemical reactions. It interacts with enzymes, proteins, and other biomolecules. For instance, it can be used as a reagent to synthesize spiro[indoline-3,4′-pyrano[2,3-c]pyrazole derivatives via a one-pot reaction with isatins, malononitrile, and hydrazine hydrate . It can also react with various aldehydes and primary amines to produce 2,3-dioxo-4-carboxy-5-substituted pyrrolidines .
Cellular Effects
The effects of this compound on cells and cellular processes are complex and multifaceted. It influences cell function, including impacts on cell signaling pathways, gene expression, and cellular metabolism
Molecular Mechanism
The molecular mechanism of this compound involves its interactions with biomolecules at the molecular level. It exerts its effects through binding interactions with biomolecules, enzyme inhibition or activation, and changes in gene expression
Dosage Effects in Animal Models
The effects of this compound vary with different dosages in animal models
Metabolic Pathways
This compound is involved in several metabolic pathways. It interacts with enzymes and cofactors, and can affect metabolic flux or metabolite levels
Transport and Distribution
The transport and distribution of this compound within cells and tissues involve interactions with transporters or binding proteins
Méthodes De Préparation
Synthetic Routes and Reaction Conditions: Diethyl oxalacetate can be synthesized through the esterification of oxaloacetic acid with ethanol in the presence of an acid catalyst. Another method involves the reaction of diethyl oxalate with ethyl acetate in the presence of sodium ethoxide. The reaction conditions typically include maintaining a temperature range of 0°C to 40°C and stirring the mixture for several hours .
Industrial Production Methods: In industrial settings, this compound is produced by adding sodium ethoxide to a mixture of diethyl oxalate and ethyl acetate. The reaction mixture is then heated to around 70°C to 80°C for a specified period, followed by cooling and purification to obtain the final product .
Analyse Des Réactions Chimiques
Types of Reactions: Diethyl oxalacetate undergoes various chemical reactions, including:
Oxidation: It can be oxidized to form oxaloacetic acid.
Reduction: It can be reduced to form diethyl malate.
Substitution: It can participate in nucleophilic substitution reactions to form various derivatives.
Common Reagents and Conditions:
Oxidation: Common oxidizing agents include potassium permanganate and chromium trioxide.
Reduction: Reducing agents such as sodium borohydride and lithium aluminum hydride are used.
Substitution: Reagents like alkyl halides and amines are commonly used under basic or acidic conditions.
Major Products:
Oxidation: Oxaloacetic acid.
Reduction: Diethyl malate.
Substitution: Various substituted oxalacetates and pyrazole derivatives
Applications De Recherche Scientifique
Diethyl oxalacetate has a wide range of applications in scientific research:
Biology: It is used in studies related to metabolic pathways and enzyme reactions.
Medicine: It serves as an intermediate in the synthesis of pharmaceutical compounds.
Industry: It is used in the production of dyes, herbicides, and other industrial chemicals
Comparaison Avec Des Composés Similaires
Diethyl malonate: Another diethyl ester used in organic synthesis.
Diethyl oxalpropionate: Similar in structure and used in similar reactions.
Diethyl oxalate: Used as a reagent in organic synthesis.
Uniqueness: Diethyl oxalacetate is unique due to its specific role in the citric acid cycle and its ability to participate in a wide range of chemical reactions. Its versatility in synthetic applications and its involvement in metabolic pathways make it distinct from other similar compounds .
Propriétés
IUPAC Name |
diethyl 2-oxobutanedioate | |
---|---|---|
Source | PubChem | |
URL | https://pubchem.ncbi.nlm.nih.gov | |
Description | Data deposited in or computed by PubChem | |
InChI |
InChI=1S/C8H12O5/c1-3-12-7(10)5-6(9)8(11)13-4-2/h3-5H2,1-2H3 | |
Source | PubChem | |
URL | https://pubchem.ncbi.nlm.nih.gov | |
Description | Data deposited in or computed by PubChem | |
InChI Key |
JDXYSCUOABNLIR-UHFFFAOYSA-N | |
Source | PubChem | |
URL | https://pubchem.ncbi.nlm.nih.gov | |
Description | Data deposited in or computed by PubChem | |
Canonical SMILES |
CCOC(=O)CC(=O)C(=O)OCC | |
Source | PubChem | |
URL | https://pubchem.ncbi.nlm.nih.gov | |
Description | Data deposited in or computed by PubChem | |
Molecular Formula |
C8H12O5 | |
Source | PubChem | |
URL | https://pubchem.ncbi.nlm.nih.gov | |
Description | Data deposited in or computed by PubChem | |
DSSTOX Substance ID |
DTXSID60148351 | |
Record name | Diethyl 2-oxobutanedioate | |
Source | EPA DSSTox | |
URL | https://comptox.epa.gov/dashboard/DTXSID60148351 | |
Description | DSSTox provides a high quality public chemistry resource for supporting improved predictive toxicology. | |
Molecular Weight |
188.18 g/mol | |
Source | PubChem | |
URL | https://pubchem.ncbi.nlm.nih.gov | |
Description | Data deposited in or computed by PubChem | |
Physical Description |
Oily liquid; [Merck Index] Clear colorless to slightly yellow liquid; [TCI America MSDS] | |
Record name | Diethyl oxalacetate | |
Source | Haz-Map, Information on Hazardous Chemicals and Occupational Diseases | |
URL | https://haz-map.com/Agents/20589 | |
Description | Haz-Map® is an occupational health database designed for health and safety professionals and for consumers seeking information about the adverse effects of workplace exposures to chemical and biological agents. | |
Explanation | Copyright (c) 2022 Haz-Map(R). All rights reserved. Unless otherwise indicated, all materials from Haz-Map are copyrighted by Haz-Map(R). No part of these materials, either text or image may be used for any purpose other than for personal use. Therefore, reproduction, modification, storage in a retrieval system or retransmission, in any form or by any means, electronic, mechanical or otherwise, for reasons other than personal use, is strictly prohibited without prior written permission. | |
CAS No. |
108-56-5 | |
Record name | Diethyl oxalacetate | |
Source | CAS Common Chemistry | |
URL | https://commonchemistry.cas.org/detail?cas_rn=108-56-5 | |
Description | CAS Common Chemistry is an open community resource for accessing chemical information. Nearly 500,000 chemical substances from CAS REGISTRY cover areas of community interest, including common and frequently regulated chemicals, and those relevant to high school and undergraduate chemistry classes. This chemical information, curated by our expert scientists, is provided in alignment with our mission as a division of the American Chemical Society. | |
Explanation | The data from CAS Common Chemistry is provided under a CC-BY-NC 4.0 license, unless otherwise stated. | |
Record name | Ethyl oxalacetate | |
Source | ChemIDplus | |
URL | https://pubchem.ncbi.nlm.nih.gov/substance/?source=chemidplus&sourceid=0000108565 | |
Description | ChemIDplus is a free, web search system that provides access to the structure and nomenclature authority files used for the identification of chemical substances cited in National Library of Medicine (NLM) databases, including the TOXNET system. | |
Record name | Diethyl oxalacetate | |
Source | DTP/NCI | |
URL | https://dtp.cancer.gov/dtpstandard/servlet/dwindex?searchtype=NSC&outputformat=html&searchlist=68476 | |
Description | The NCI Development Therapeutics Program (DTP) provides services and resources to the academic and private-sector research communities worldwide to facilitate the discovery and development of new cancer therapeutic agents. | |
Explanation | Unless otherwise indicated, all text within NCI products is free of copyright and may be reused without our permission. Credit the National Cancer Institute as the source. | |
Record name | Diethyl 2-oxobutanedioate | |
Source | EPA DSSTox | |
URL | https://comptox.epa.gov/dashboard/DTXSID60148351 | |
Description | DSSTox provides a high quality public chemistry resource for supporting improved predictive toxicology. | |
Record name | Diethyl oxalacetate | |
Source | European Chemicals Agency (ECHA) | |
URL | https://echa.europa.eu/substance-information/-/substanceinfo/100.003.268 | |
Description | The European Chemicals Agency (ECHA) is an agency of the European Union which is the driving force among regulatory authorities in implementing the EU's groundbreaking chemicals legislation for the benefit of human health and the environment as well as for innovation and competitiveness. | |
Explanation | Use of the information, documents and data from the ECHA website is subject to the terms and conditions of this Legal Notice, and subject to other binding limitations provided for under applicable law, the information, documents and data made available on the ECHA website may be reproduced, distributed and/or used, totally or in part, for non-commercial purposes provided that ECHA is acknowledged as the source: "Source: European Chemicals Agency, http://echa.europa.eu/". Such acknowledgement must be included in each copy of the material. ECHA permits and encourages organisations and individuals to create links to the ECHA website under the following cumulative conditions: Links can only be made to webpages that provide a link to the Legal Notice page. | |
Record name | DIETHYL OXALACETATE | |
Source | FDA Global Substance Registration System (GSRS) | |
URL | https://gsrs.ncats.nih.gov/ginas/app/beta/substances/15S56468G7 | |
Description | The FDA Global Substance Registration System (GSRS) enables the efficient and accurate exchange of information on what substances are in regulated products. Instead of relying on names, which vary across regulatory domains, countries, and regions, the GSRS knowledge base makes it possible for substances to be defined by standardized, scientific descriptions. | |
Explanation | Unless otherwise noted, the contents of the FDA website (www.fda.gov), both text and graphics, are not copyrighted. They are in the public domain and may be republished, reprinted and otherwise used freely by anyone without the need to obtain permission from FDA. Credit to the U.S. Food and Drug Administration as the source is appreciated but not required. | |
Synthesis routes and methods I
Procedure details
Synthesis routes and methods II
Procedure details
Synthesis routes and methods III
Procedure details
Retrosynthesis Analysis
AI-Powered Synthesis Planning: Our tool employs the Template_relevance Pistachio, Template_relevance Bkms_metabolic, Template_relevance Pistachio_ringbreaker, Template_relevance Reaxys, Template_relevance Reaxys_biocatalysis model, leveraging a vast database of chemical reactions to predict feasible synthetic routes.
One-Step Synthesis Focus: Specifically designed for one-step synthesis, it provides concise and direct routes for your target compounds, streamlining the synthesis process.
Accurate Predictions: Utilizing the extensive PISTACHIO, BKMS_METABOLIC, PISTACHIO_RINGBREAKER, REAXYS, REAXYS_BIOCATALYSIS database, our tool offers high-accuracy predictions, reflecting the latest in chemical research and data.
Strategy Settings
Precursor scoring | Relevance Heuristic |
---|---|
Min. plausibility | 0.01 |
Model | Template_relevance |
Template Set | Pistachio/Bkms_metabolic/Pistachio_ringbreaker/Reaxys/Reaxys_biocatalysis |
Top-N result to add to graph | 6 |
Feasible Synthetic Routes
Avertissement et informations sur les produits de recherche in vitro
Veuillez noter que tous les articles et informations sur les produits présentés sur BenchChem sont destinés uniquement à des fins informatives. Les produits disponibles à l'achat sur BenchChem sont spécifiquement conçus pour des études in vitro, qui sont réalisées en dehors des organismes vivants. Les études in vitro, dérivées du terme latin "in verre", impliquent des expériences réalisées dans des environnements de laboratoire contrôlés à l'aide de cellules ou de tissus. Il est important de noter que ces produits ne sont pas classés comme médicaments et n'ont pas reçu l'approbation de la FDA pour la prévention, le traitement ou la guérison de toute condition médicale, affection ou maladie. Nous devons souligner que toute forme d'introduction corporelle de ces produits chez les humains ou les animaux est strictement interdite par la loi. Il est essentiel de respecter ces directives pour assurer la conformité aux normes légales et éthiques en matière de recherche et d'expérimentation.