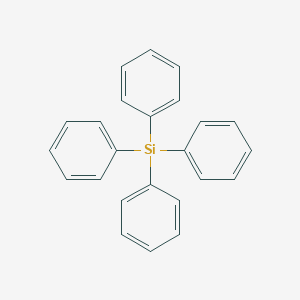
Tétraphénylsilane
Vue d'ensemble
Description
Tetraphenylsilane is an organosilicon compound with the chemical formula ( \text{C}{24}\text{H}{20}\text{Si} ). It is characterized by a silicon atom bonded to four phenyl groups. This compound is known for its stability and unique properties, making it a valuable material in various scientific and industrial applications.
Applications De Recherche Scientifique
Tetraphenylsilane has a wide range of applications in scientific research:
Mécanisme D'action
Target of Action
Tetraphenylsilane (TPSi) is primarily used in the field of advanced lithography and optoelectronics . Its primary targets are the materials used in these fields, such as resist materials in lithography and host materials in organic light-emitting diodes (OLEDs) .
Mode of Action
Tetraphenylsilane interacts with its targets by contributing to the physical properties of the materials. For instance, in lithography, TPSi derivatives are used as resist materials, where they undergo changes under the influence of an electron beam . In OLEDs, TPSi derivatives are used as host materials, where they facilitate hole injection from the nearby hole-transporting layer .
Biochemical Pathways
In lithography, the TPSi derivatives undergo changes under the electron beam, affecting the contrast, pattern blur, and density of bridge defect . In OLEDs, the introduction of spiro-annulated triphenylamine/carbazole moieties on TPSi increases the HOMO energy levels, facilitating hole injection .
Pharmacokinetics
Its properties such as solubility, film-forming ability, and thermal stability are crucial for its application in advanced lithography . In terms of bioavailability, it is worth noting that TPSi is classified as having low GI absorption and is a substrate of P-gp .
Result of Action
The action of TPSi results in significant changes in the materials it interacts with. In lithography, the use of TPSi derivatives as resist materials can lead to changes in contrast, pattern blur, and the density of bridge defect . In OLEDs, TPSi derivatives can enhance the HOMO energy levels and glass transition temperatures without lowering triplet energy .
Action Environment
The action of TPSi is influenced by environmental factors such as temperature and the presence of other chemicals. For instance, the thermal stability of TPSi derivatives is crucial for their application in advanced lithography . Additionally, the safety data sheet for TPSi suggests that it should be stored in an inert atmosphere at room temperature .
Méthodes De Préparation
Synthetic Routes and Reaction Conditions: Tetraphenylsilane can be synthesized through several methods:
Reaction of Phenylmagnesium Bromide with Tetrachlorosilane: This method involves the reaction of phenylmagnesium bromide with tetrachlorosilane under controlled conditions to yield tetraphenylsilane.
Reaction of Phenyllithium with Alkoxysilanes: Another synthetic route involves the reaction of phenyllithium with alkoxysilanes, resulting in the formation of tetraphenylsilane.
Industrial Production Methods: In industrial settings, tetraphenylsilane is typically produced using large-scale reactions involving phenylmagnesium bromide and tetrachlorosilane. The reaction is carried out in an inert atmosphere to prevent unwanted side reactions and to ensure high yield and purity of the product .
Analyse Des Réactions Chimiques
Types of Reactions: Tetraphenylsilane undergoes various chemical reactions, including:
Substitution Reactions: Tetraphenylsilane can participate in substitution reactions where one or more phenyl groups are replaced by other substituents.
Oxidation and Reduction Reactions: Although tetraphenylsilane is relatively stable, it can undergo oxidation and reduction reactions under specific conditions.
Common Reagents and Conditions:
Substitution Reactions: Common reagents include halogens and organometallic compounds.
Oxidation and Reduction Reactions: Oxidizing agents such as hydrogen peroxide and reducing agents like lithium aluminum hydride are used in these reactions.
Major Products Formed: The major products formed from these reactions depend on the specific reagents and conditions used. For example, substitution reactions can yield various substituted silanes, while oxidation reactions may produce silanols .
Comparaison Avec Des Composés Similaires
- Tetraphenylmethane
- Tetraphenylgermane
- Tetraphenylstannane
Tetraphenylsilane’s unique combination of stability and reactivity makes it a versatile and valuable compound in various fields of science and industry.
Activité Biologique
Tetraphenylsilane (TPS) is an organosilicon compound that has garnered attention in various fields, including materials science and medicinal chemistry. This article explores the biological activity of TPS, focusing on its synthesis, photophysical properties, and potential applications in biomedical contexts.
Overview of Tetraphenylsilane
Tetraphenylsilane is a silicon-based compound characterized by four phenyl groups attached to a silicon atom. Its unique structure imparts significant electronic properties, making it a subject of interest in the development of advanced materials, particularly in organic light-emitting diodes (OLEDs) and photonic devices. Moreover, its biological activity has been investigated for potential therapeutic applications.
Synthesis and Characterization
The synthesis of TPS typically involves methods such as hydrosilylation and Friedel-Crafts reactions. For instance, one common synthetic route includes the reaction of phenylmagnesium bromide with dichlorosilane, followed by hydrolysis to yield TPS. The characterization of TPS can be performed using techniques like nuclear magnetic resonance (NMR) spectroscopy and mass spectrometry, which confirm the structure and purity of the compound.
Anticancer Properties
Recent studies have demonstrated that TPS exhibits anticancer activity through various mechanisms:
- Drug Delivery Systems : TPS has been incorporated into micellar systems that enhance drug solubility and bioavailability. These drug-loaded micelles show significant cytotoxic effects against cancer cell lines while maintaining low toxicity in normal cells . The pH-responsive nature of these micelles allows for effective drug release in acidic tumor microenvironments.
- Photophysical Properties : TPS derivatives have shown promising results in photothermal therapy. The ability of TPS to absorb light and convert it into heat can be utilized to selectively destroy cancer cells when exposed to specific wavelengths .
The mechanisms underlying the biological activity of TPS are multifaceted:
- Reactive Oxygen Species (ROS) Generation : Upon excitation, TPS can generate ROS, which are known to induce apoptosis in cancer cells. This property is particularly useful in photodynamic therapy (PDT), where light-activated compounds selectively target tumor cells .
- Cellular Uptake : Studies indicate that TPS can enhance cellular uptake due to its lipophilic nature, facilitating better interaction with cellular membranes and improving therapeutic efficacy .
Case Studies
- In Vitro Studies : A study evaluated the cytotoxic effects of TPS-loaded micelles on various cancer cell lines, including breast and lung cancer. Results indicated a dose-dependent decrease in cell viability, with TPS demonstrating higher efficacy compared to conventional chemotherapeutics .
- In Vivo Models : In animal models, TPS derivatives were tested for their ability to inhibit tumor growth. The results showed a significant reduction in tumor size compared to control groups, highlighting the potential of TPS as an effective anticancer agent .
Photophysical Properties
The photophysical properties of TPS contribute significantly to its biological applications:
- Fluorescence Emission : TPS exhibits strong fluorescence properties with quantum yields suitable for applications in imaging and diagnostics . The photoluminescence maximum observed at 508 nm indicates its potential use as a fluorescent marker in biological systems.
- Thermally Activated Delayed Fluorescence (TADF) : Recent advancements have shown that TPS derivatives can exhibit TADF characteristics, enhancing their applicability in OLED technology while also providing insights into their behavior in biological contexts .
Propriétés
IUPAC Name |
tetraphenylsilane | |
---|---|---|
Source | PubChem | |
URL | https://pubchem.ncbi.nlm.nih.gov | |
Description | Data deposited in or computed by PubChem | |
InChI |
InChI=1S/C24H20Si/c1-5-13-21(14-6-1)25(22-15-7-2-8-16-22,23-17-9-3-10-18-23)24-19-11-4-12-20-24/h1-20H | |
Source | PubChem | |
URL | https://pubchem.ncbi.nlm.nih.gov | |
Description | Data deposited in or computed by PubChem | |
InChI Key |
JLAVCPKULITDHO-UHFFFAOYSA-N | |
Source | PubChem | |
URL | https://pubchem.ncbi.nlm.nih.gov | |
Description | Data deposited in or computed by PubChem | |
Canonical SMILES |
C1=CC=C(C=C1)[Si](C2=CC=CC=C2)(C3=CC=CC=C3)C4=CC=CC=C4 | |
Source | PubChem | |
URL | https://pubchem.ncbi.nlm.nih.gov | |
Description | Data deposited in or computed by PubChem | |
Molecular Formula |
C24H20Si | |
Source | PubChem | |
URL | https://pubchem.ncbi.nlm.nih.gov | |
Description | Data deposited in or computed by PubChem | |
DSSTOX Substance ID |
DTXSID2061430 | |
Record name | Tetraphenylsilane | |
Source | EPA DSSTox | |
URL | https://comptox.epa.gov/dashboard/DTXSID2061430 | |
Description | DSSTox provides a high quality public chemistry resource for supporting improved predictive toxicology. | |
Molecular Weight |
336.5 g/mol | |
Source | PubChem | |
URL | https://pubchem.ncbi.nlm.nih.gov | |
Description | Data deposited in or computed by PubChem | |
CAS No. |
1048-08-4 | |
Record name | 1,1′,1′′,1′′′-Silanetetrayltetrakis[benzene] | |
Source | CAS Common Chemistry | |
URL | https://commonchemistry.cas.org/detail?cas_rn=1048-08-4 | |
Description | CAS Common Chemistry is an open community resource for accessing chemical information. Nearly 500,000 chemical substances from CAS REGISTRY cover areas of community interest, including common and frequently regulated chemicals, and those relevant to high school and undergraduate chemistry classes. This chemical information, curated by our expert scientists, is provided in alignment with our mission as a division of the American Chemical Society. | |
Explanation | The data from CAS Common Chemistry is provided under a CC-BY-NC 4.0 license, unless otherwise stated. | |
Record name | Tetraphenylsilane | |
Source | ChemIDplus | |
URL | https://pubchem.ncbi.nlm.nih.gov/substance/?source=chemidplus&sourceid=0001048084 | |
Description | ChemIDplus is a free, web search system that provides access to the structure and nomenclature authority files used for the identification of chemical substances cited in National Library of Medicine (NLM) databases, including the TOXNET system. | |
Record name | Tetraphenylsilane | |
Source | DTP/NCI | |
URL | https://dtp.cancer.gov/dtpstandard/servlet/dwindex?searchtype=NSC&outputformat=html&searchlist=33014 | |
Description | The NCI Development Therapeutics Program (DTP) provides services and resources to the academic and private-sector research communities worldwide to facilitate the discovery and development of new cancer therapeutic agents. | |
Explanation | Unless otherwise indicated, all text within NCI products is free of copyright and may be reused without our permission. Credit the National Cancer Institute as the source. | |
Record name | Benzene, 1,1',1'',1'''-silanetetrayltetrakis- | |
Source | EPA Chemicals under the TSCA | |
URL | https://www.epa.gov/chemicals-under-tsca | |
Description | EPA Chemicals under the Toxic Substances Control Act (TSCA) collection contains information on chemicals and their regulations under TSCA, including non-confidential content from the TSCA Chemical Substance Inventory and Chemical Data Reporting. | |
Record name | Tetraphenylsilane | |
Source | EPA DSSTox | |
URL | https://comptox.epa.gov/dashboard/DTXSID2061430 | |
Description | DSSTox provides a high quality public chemistry resource for supporting improved predictive toxicology. | |
Record name | Tetraphenylsilane | |
Source | European Chemicals Agency (ECHA) | |
URL | https://echa.europa.eu/substance-information/-/substanceinfo/100.012.620 | |
Description | The European Chemicals Agency (ECHA) is an agency of the European Union which is the driving force among regulatory authorities in implementing the EU's groundbreaking chemicals legislation for the benefit of human health and the environment as well as for innovation and competitiveness. | |
Explanation | Use of the information, documents and data from the ECHA website is subject to the terms and conditions of this Legal Notice, and subject to other binding limitations provided for under applicable law, the information, documents and data made available on the ECHA website may be reproduced, distributed and/or used, totally or in part, for non-commercial purposes provided that ECHA is acknowledged as the source: "Source: European Chemicals Agency, http://echa.europa.eu/". Such acknowledgement must be included in each copy of the material. ECHA permits and encourages organisations and individuals to create links to the ECHA website under the following cumulative conditions: Links can only be made to webpages that provide a link to the Legal Notice page. | |
Record name | TETRAPHENYLSILICON | |
Source | FDA Global Substance Registration System (GSRS) | |
URL | https://gsrs.ncats.nih.gov/ginas/app/beta/substances/9XSD5J49CO | |
Description | The FDA Global Substance Registration System (GSRS) enables the efficient and accurate exchange of information on what substances are in regulated products. Instead of relying on names, which vary across regulatory domains, countries, and regions, the GSRS knowledge base makes it possible for substances to be defined by standardized, scientific descriptions. | |
Explanation | Unless otherwise noted, the contents of the FDA website (www.fda.gov), both text and graphics, are not copyrighted. They are in the public domain and may be republished, reprinted and otherwise used freely by anyone without the need to obtain permission from FDA. Credit to the U.S. Food and Drug Administration as the source is appreciated but not required. | |
Retrosynthesis Analysis
AI-Powered Synthesis Planning: Our tool employs the Template_relevance Pistachio, Template_relevance Bkms_metabolic, Template_relevance Pistachio_ringbreaker, Template_relevance Reaxys, Template_relevance Reaxys_biocatalysis model, leveraging a vast database of chemical reactions to predict feasible synthetic routes.
One-Step Synthesis Focus: Specifically designed for one-step synthesis, it provides concise and direct routes for your target compounds, streamlining the synthesis process.
Accurate Predictions: Utilizing the extensive PISTACHIO, BKMS_METABOLIC, PISTACHIO_RINGBREAKER, REAXYS, REAXYS_BIOCATALYSIS database, our tool offers high-accuracy predictions, reflecting the latest in chemical research and data.
Strategy Settings
Precursor scoring | Relevance Heuristic |
---|---|
Min. plausibility | 0.01 |
Model | Template_relevance |
Template Set | Pistachio/Bkms_metabolic/Pistachio_ringbreaker/Reaxys/Reaxys_biocatalysis |
Top-N result to add to graph | 6 |
Feasible Synthetic Routes
Q1: What makes tetraphenylsilane suitable for OLED applications?
A1: Tetraphenylsilane derivatives are attractive for OLEDs due to several key properties:
- Wide bandgap: This allows for the design of blue-emitting materials, a crucial color component in OLED displays. [, , , , , ]
- High triplet energy: This is essential for hosting phosphorescent emitters, which can achieve 100% internal quantum efficiency. [, , , , ]
- Good thermal and morphological stability: This ensures device longevity and consistent performance. [, , , , ]
- Solubility and processability: Tetraphenylsilane derivatives can be readily synthesized and processed from solution, enabling large-area and low-cost fabrication techniques. [, , , , ]
Q2: How does the structure of tetraphenylsilane contribute to its amorphous nature in thin films?
A2: The tetrahedral geometry of the central silicon atom, combined with the bulky phenyl groups, hinders efficient packing and crystallization. This inherent steric hindrance favors the formation of amorphous films, which is advantageous for OLEDs as it prevents grain boundaries and improves device uniformity. [, , , , ]
Q3: Beyond OLEDs, what other applications utilize tetraphenylsilane's unique properties?
A3: Besides OLEDs, tetraphenylsilane derivatives are explored in diverse applications:
- Porous materials: Tetraphenylsilane can act as a building block in porous materials, where its rigid structure helps create permanent porosity. The phenyl rings can be further functionalized to tune the material's properties. [, , ]
- Molecular glass resists: Tetraphenylsilane derivatives have been investigated as potential resist materials for advanced lithographic techniques like electron beam lithography due to their high resolution and etch selectivity. []
- Sensing materials: Conjugated microporous polymers incorporating tetraphenylsilane units have shown promise for nitroaromatic detection in aqueous media due to their high sensitivity and selectivity. []
Q4: What is the molecular formula and weight of tetraphenylsilane?
A4: The molecular formula is Si(C6H5)4, and its molecular weight is 308.48 g/mol.
Q5: How does the introduction of different substituents onto the tetraphenylsilane core affect its properties?
A5: Incorporating various functional groups into the tetraphenylsilane scaffold significantly influences the material's properties, making it suitable for a wider range of applications:
- Electron-donating groups (carbazole): Enhance hole-transport properties and increase the HOMO energy level, beneficial for balanced charge transport in OLEDs. [, , , , , ]
- Electron-withdrawing groups (phosphine oxide, phosphonate, pyridine): Improve electron-transport properties and lower the LUMO energy level, facilitating electron injection in OLEDs. [, , , , ]
- Bulky groups (tert-butyl): Enhance solubility, increase glass transition temperatures, and reduce aggregation-caused quenching, leading to improved device performance. [, ]
- Fluorescent moieties (TPAOXD): Lead to the development of bright blue-emitting molecular glasses for OLED applications. [, ]
Q6: How does the linkage position of substituents on the phenyl rings of tetraphenylsilane affect the material properties?
A6: Research has shown that even subtle changes in the linkage position can significantly impact the material's properties. For example, in pyridine-containing tetraphenylsilane hosts for OLEDs, the nitrogen atom orientation of the pyridine ring significantly influences the LUMO energy levels and electron-transport characteristics, leading to variations in device performance. []
Q7: What are the typical thermal and oxidative degradation pathways observed in tetraphenylsilane-containing polymers?
A7: Studies have revealed multiple degradation pathways, including:
- Cleavage of Si-phenyl bonds: This can occur at elevated temperatures, leading to the formation of volatile benzene and other byproducts. [, , ]
- Oxidation of phenyl rings: This process can lead to the formation of phenolic compounds and ultimately result in material degradation. [, ]
- Formation of Si-O-Si bonds: This crosslinking reaction can occur at high temperatures, contributing to an increase in the material's glass transition temperature and potentially affecting its processability. []
Q8: How can the thermal and oxidative stability of tetraphenylsilane-containing materials be improved?
A8: Several strategies can be employed to enhance stability:
- Introducing bulky substituents: This can hinder access to reactive sites and improve the material's resistance to thermal and oxidative degradation. []
- Using appropriate curing conditions: Optimizing the curing temperature and catalyst concentration can lead to more complete polymerization and a more stable network structure. []
Q9: What are the known environmental impacts of tetraphenylsilane and its derivatives?
A9: While specific ecotoxicological data for tetraphenylsilane and its derivatives are limited in the provided literature, it is essential to consider:
Q10: How is computational chemistry being used to understand and design novel tetraphenylsilane-based materials?
A10: Computational methods like Density Functional Theory (DFT) play a crucial role in:
- Predicting electronic properties: Calculating the HOMO and LUMO energy levels helps in designing materials with targeted optoelectronic properties. [, , ]
- Understanding structure-property relationships: Simulations can elucidate how different substituents and their positions affect the material's conformation, electronic properties, and overall performance. [, , ]
- Predicting hyperfine interactions: DFT calculations have been used to examine the hyperfine interactions of muonium in tetraphenylsilane, providing valuable insights into the material's electronic structure. []
Q11: What are some future research directions for tetraphenylsilane-based materials?
A11: Promising avenues for future research include:
- Developing deep-blue and ultra-violet emitting materials: Expanding the color palette of tetraphenylsilane-based emitters for next-generation OLED displays. [, ]
- Enhancing the efficiency and stability of blue OLEDs: Addressing challenges related to efficiency roll-off and device lifetime. [, , ]
- Exploring new applications beyond optoelectronics: Leveraging the unique structural and electronic properties of tetraphenylsilane in areas like sensing, catalysis, and biomedicine. [, , ]
Avertissement et informations sur les produits de recherche in vitro
Veuillez noter que tous les articles et informations sur les produits présentés sur BenchChem sont destinés uniquement à des fins informatives. Les produits disponibles à l'achat sur BenchChem sont spécifiquement conçus pour des études in vitro, qui sont réalisées en dehors des organismes vivants. Les études in vitro, dérivées du terme latin "in verre", impliquent des expériences réalisées dans des environnements de laboratoire contrôlés à l'aide de cellules ou de tissus. Il est important de noter que ces produits ne sont pas classés comme médicaments et n'ont pas reçu l'approbation de la FDA pour la prévention, le traitement ou la guérison de toute condition médicale, affection ou maladie. Nous devons souligner que toute forme d'introduction corporelle de ces produits chez les humains ou les animaux est strictement interdite par la loi. Il est essentiel de respecter ces directives pour assurer la conformité aux normes légales et éthiques en matière de recherche et d'expérimentation.