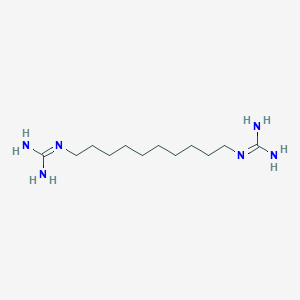
Synthalin A
Vue d'ensemble
Description
Synthalin A (decamethylenediguanidine) is a biguanide derivative first synthesized in the 1920s as an oral hypoglycemic agent for diabetes mellitus. Derived from guanidine, it was developed to mimic insulin's glucose-lowering effects while avoiding the need for injections . Early studies proposed that this compound reduced blood glucose by suppressing hepatic glycogenolysis and enhancing endogenous insulin secretion . However, its clinical use was overshadowed by insulin's discovery and later discontinued due to hepatotoxicity risks, including jaundice and liver atrophy .
Méthodes De Préparation
Voies de synthèse et conditions réactionnelles
Synthalin est synthétisé en créant une chaîne polyméthylène avec un groupe guanidine à chaque extrémité. La synthèse implique la réaction de la diamine décaméthylène avec la guanidine pour former le dérivé diguanidine. Les conditions réactionnelles impliquent généralement l'utilisation de solvants et de températures contrôlées pour assurer la formation du produit désiré .
Méthodes de production industrielle
La production industrielle de Synthalin aurait impliqué une synthèse à grande échelle utilisant des méthodes similaires à celles décrites ci-dessus, mais avec des contrôles plus stricts et des étapes de purification pour assurer l'élimination des sous-produits toxiques. La production aurait été effectuée dans des installations spécialisées équipées pour manipuler les produits chimiques et les conditions réactionnelles nécessaires à la synthèse .
Analyse Des Réactions Chimiques
Types de réactions
Synthalin subit plusieurs types de réactions chimiques, notamment :
Oxydation : Synthalin peut être oxydé pour former divers produits d'oxydation.
Réduction : Le composé peut être réduit dans des conditions spécifiques pour produire des formes réduites.
Substitution : Synthalin peut subir des réactions de substitution où un ou plusieurs de ses groupes fonctionnels sont remplacés par d'autres groupes.
Réactifs et conditions courants
Oxydation : Des agents oxydants courants tels que le permanganate de potassium ou le peroxyde d'hydrogène peuvent être utilisés.
Réduction : Des agents réducteurs comme le borohydrure de sodium ou l'hydrure de lithium et d'aluminium sont généralement utilisés.
Substitution : Divers réactifs en fonction de la substitution désirée, tels que des halogènes ou des agents alkylants.
Principaux produits formés
Les principaux produits formés à partir de ces réactions dépendent des conditions spécifiques et des réactifs utilisés. Par exemple, l'oxydation peut produire des dérivés oxydés, tandis que la réduction peut produire des formes réduites de Synthalin .
Applications de la recherche scientifique
Synthalin a fait l'objet de nombreuses recherches, notamment dans les domaines de la chimie, de la biologie et de la médecine. Voici quelques-unes de ses applications :
Chimie : this compound été étudié pour ses propriétés chimiques uniques et son potentiel comme précurseur d'autres composés.
Biologie : La recherche s'est concentrée sur ses effets sur les systèmes biologiques, en particulier ses effets hypoglycémiques.
Médecine : Synthalin était initialement utilisé comme médicament antidiabétique en raison de ses propriétés similaires à celles de l'insuline.
Mécanisme d'action
Synthalin exerce ses effets principalement en ciblant les cellules alpha pancréatiques, ce qui conduit à l'épuisement de ces cellules et à une réduction de la sécrétion de glucagon. Cela entraîne une diminution du taux de glucose sanguin. Le mécanisme du composé implique des dommages aux cellules alpha tout en épargnant les cellules bêta, offrant ainsi un moyen de diminuer le glucose sanguin en réduisant la sécrétion de glucagon dans les états non diabétiques et diabétiques .
Applications De Recherche Scientifique
Introduction to Synthalin A
This compound (Syn A) is a guanidine derivative that was initially explored in the 1920s and 1930s for its potential as an anti-diabetic agent. It was primarily studied for its ability to suppress glucagon secretion from pancreatic alpha-cells, thereby lowering blood glucose levels. Although interest in Syn A waned with the advent of insulin therapy, recent evaluations of its historical applications may provide insights into contemporary diabetes treatment strategies.
Historical Context and Mechanism of Action
This compound was developed as a potential treatment for diabetes by targeting the glucagon-producing alpha-cells in the pancreas. Glucagon plays a crucial role in increasing blood glucose levels, counteracting the effects of insulin. Early studies indicated that Syn A could induce morphological changes in alpha-cells, leading to their dysfunction and subsequent reduction of glucagon secretion. This biphasic action initially caused transient hyperglycemia followed by hypoglycemia due to the depletion of glycogen stores and exhaustion of alpha-cell function .
Key Findings from Historical Research
- Hypoglycemic Effects : this compound demonstrated the ability to lower blood glucose levels in both animal models and human subjects when administered in various forms, including tablets and injectables .
- Cellular Impact : Studies revealed that Syn A induced hydropic changes and cytoplasmic vacuolation in pancreatic alpha-cells, suggesting a mechanism of action that involved damage to these cells while sparing beta-cells .
- Safety Concerns : Reports of adverse effects such as proteinuria, hematuria, and digestive complications raised concerns about the safety of this compound, leading to its discontinuation as a diabetes treatment by the late 1930s .
Applications Beyond Diabetes
Despite its decline in use for diabetes management, this compound has been investigated for other therapeutic applications:
Anti-Trypanosomal Activity
Research indicated that Synthalin and its derivatives could effectively target trypanosomes, which require glucose for reproduction. This led to the development of modified compounds within the diamidine class, such as pentamidine, which remains in use today for treating trypanosomiasis and other protozoal infections .
Summary of Applications
Application Area | Description |
---|---|
Diabetes Management | Suppression of glucagon secretion; historical interest for lowering blood glucose levels. |
Anti-Trypanosomal | Effective against trypanosomiasis; led to development of pentamidine and related compounds. |
Case Study 1: Historical Diabetes Treatment
In early clinical trials, this compound was administered to patients with diabetes, demonstrating significant reductions in blood sugar levels. However, the associated risks led to its eventual withdrawal from clinical use.
Case Study 2: Anti-Trypanosomal Efficacy
Studies conducted on animal models showed that Synthalin derivatives effectively inhibited the growth of trypanosomes at doses that did not significantly affect blood glucose levels. This prompted further exploration into its chemical modifications for enhanced efficacy and safety.
Mécanisme D'action
Synthalin exerts its effects primarily by targeting pancreatic alpha-cells, leading to the depletion of these cells and a reduction in glucagon secretion. This results in lower blood glucose levels. The compound’s mechanism involves damaging alpha-cells while sparing beta-cells, thereby offering a means of decreasing blood glucose by reducing glucagon secretion in both non-diabetic and diabetic states .
Comparaison Avec Des Composés Similaires
Structural and Pharmacological Differences
The table below compares Synthalin A with contemporaneous and modern biguanides:
Key Research Findings
- This compound vs. Insulin : this compound achieved glycemic control in mild-to-moderate diabetes but required 2–3 days to take effect, unlike insulin's rapid action. In severe cases, insulin remained indispensable .
- This compound vs. Synthalin B : Synthalin B's extended alkyl chain improved tolerability but retained hepatotoxicity risks. Both compounds were phased out by the 1940s .
- This compound vs. Modern Biguanides : Unlike metformin, this compound demonstrated α-cell-specific toxicity, reducing glucagon secretion in animal models . However, its hepatotoxicity and the advent of insulin halted further development .
Clinical and Experimental Controversies
- Efficacy Variability : Studies reported conflicting results—e.g., this compound reduced fasting glucose by 15.5% in some trials but increased it by 10.25% in others, likely due to dietary inconsistencies .
- Toxicity Debate : While some clinicians reported safe use with monitored dosing , others observed hepatic damage in 20% of long-term users .
Mechanistic Insights and Modern Relevance
This compound's dual action—suppressing hepatic glucose output and glucagon secretion—aligns with contemporary recognition of diabetes as a dual hormone disorder (insulin deficiency + glucagon excess) . Its α-cell toxicity, observed in avian and mammalian models , offers a historical precedent for modern glucagon-targeting therapies. However, its toxicity profile underscores why metformin, with its safer AMPK-mediated mechanism, became the gold-standard biguanide .
Activité Biologique
Synthalin A (decamethylenediguanidine) is a compound that was explored primarily in the 1920s and 1930s as an oral anti-diabetic agent. Its biological activity is characterized by its hypoglycemic effects, particularly through its action on pancreatic alpha-cells, but it also presents significant toxicity issues. This article delves into the biological activity of this compound, examining its mechanisms, effects on various biological systems, and safety concerns supported by case studies and research findings.
This compound acts primarily by targeting pancreatic alpha-cells, which are responsible for glucagon secretion. The compound induces morphological changes in these cells, leading to hydropic degeneration and cytoplasmic vacuolation. These alterations result in decreased glucagon secretion, thereby lowering blood glucose levels over time.
Key Findings:
- Alpha-cell Damage : Studies have shown that this compound selectively disrupts the function of alpha-cells while sparing beta-cells, which are responsible for insulin production. This selective toxicity is crucial for its hypoglycemic effects .
- Glucagon Release : Initial exposure to this compound can lead to increased glucagon release due to cellular damage, which may cause transient hyperglycemia followed by a depletion of glycogen stores and subsequent hypoglycemia .
Toxicological Profile
Despite its potential benefits in managing diabetes, this compound has a troubling safety profile. Research indicates that it can cause significant liver and kidney damage, leading to adverse effects such as proteinuria and renal complications.
Toxicity Observations:
- Liver Damage : Studies indicated extensive hepatic injury associated with this compound administration, with reports suggesting that hypoglycemic effects might be partly due to impaired gluconeogenesis .
- Renal Complications : Histological examinations revealed renal damage following treatment with this compound, raising concerns about its long-term safety in diabetic patients .
Case Studies
Several clinical observations highlight the variable efficacy and safety of this compound in treating diabetes:
- Clinical Trials in Type 1 Diabetes : In early trials conducted by Elliot Joslin in 1927, patients experienced reductions in glycosuria and some were able to decrease their insulin doses significantly. However, responses varied widely among individuals, raising questions about the consistency of its efficacy .
- Animal Studies : Experiments on various animal models demonstrated species-specific responses to this compound. For instance, guinea pig islets showed increased glucagon release and subsequent necrosis when exposed to the compound .
- Historical Context : Despite initial enthusiasm for this compound as a treatment option for diabetes, the emergence of insulin therapy and reports of toxicity led to its decline in clinical use by the late 1930s .
Comparative Data Table
The following table summarizes key findings related to the biological activity and toxicity of this compound compared to other similar compounds:
Compound | Hypoglycemic Effect | Alpha-cell Toxicity | Liver Toxicity | Kidney Toxicity |
---|---|---|---|---|
This compound | Yes | High | High | Moderate |
Synthalin B | Yes | Moderate | Moderate | Low |
Guanidine | Yes | Low | Low | Moderate |
Q & A
Basic Research Questions
Q. What is the historical significance of Synthalin A in diabetes research, and why was its study discontinued?
this compound was investigated in the 1920s–1930s as a pancreatic alpha-cell toxin to inhibit glucagon secretion, aiming to reduce hyperglycemia in diabetes. Early animal and human trials showed mixed efficacy, with some studies reporting blood sugar reductions (e.g., Series A and B) and others showing inactivity (Series C) . However, insulin’s discovery overshadowed this compound, leading to discontinued research despite its potential dual-hormone modulation (insulin deficiency and glucagon excess) .
Q. What experimental models and methodologies were used in early this compound studies?
Early studies relied on in vivo models (e.g., rabbits, chickens) to assess glycemic responses, histopathological effects (e.g., liver lesions in rabbits), and isolated islet cell analyses. Blood glucose measurements, weight changes, and patient demographics (e.g., obesity, age) were tracked, but small sample sizes (e.g., n=9 in Series C) and inconsistent protocols limited reproducibility .
Advanced Research Questions
Q. How can modern molecular techniques address historical contradictions in this compound’s efficacy data?
Contradictions (e.g., Series C vs. A/B) may stem from variability in patient subgroups (e.g., obesity, disease severity) or dosage differences. Modern approaches could include:
- Single-cell RNA sequencing to map alpha-cell specificity and off-target effects.
- Dose-response meta-analysis of historical data to identify thresholds for efficacy/toxicity.
- In vitro islet organoids to isolate this compound’s effects from confounding factors like diet .
Q. What methodological considerations are critical for designing in vivo studies on this compound’s alpha-cell specificity?
- Model selection : Use transgenic models (e.g., alpha-cell-specific reporters) to track glucagon suppression.
- Endpoint assays : Pair blood glucose measurements with glucagon ELISA and immunohistochemistry to confirm target engagement.
- Control for confounders : Standardize diets and monitor liver/kidney toxicity, given this compound’s historical hepatotoxicity .
Q. How can researchers reconcile this compound’s dual role as a potential therapeutic and toxin?
- Structure-activity relationship (SAR) studies : Modify this compound’s chemical structure to reduce off-target effects while retaining alpha-cell activity.
- Comparative toxicokinetics : Use mass spectrometry to compare metabolite profiles in responsive vs. non-responsive models .
Q. Data Contradictions and Analysis
Q. What factors explain inconsistent blood sugar responses to this compound across historical studies?
A synthesis of early data reveals key variables:
Study Series | Patient Profile | Sample Size | Blood Sugar Change | Key Limitation |
---|---|---|---|---|
Series A/B | 20% moderate cases | n=11–19 | Reduction | Small n, selection bias |
Series C | 56% severe cases | n=9 | No effect/Increase | Heterogeneous cohort |
Series D | Mixed severity | n=7 | Post-withdrawal fall | Confounding therapies |
Demographics (e.g., obesity, age) and disease severity significantly influenced outcomes. Modern studies should stratify cohorts by metabolic subtype . |
Q. Future Research Directions
Q. What gaps exist in understanding this compound’s mechanism of action, and how can they be addressed?
- Unresolved gap : this compound’s molecular targets remain unclear. Use chemoproteomics to identify binding partners in alpha-cells.
- Therapeutic synergy : Test this compound alongside insulin in dual-hormone closed-loop systems, leveraging continuous glucose monitoring (CGM) .
Q. How can researchers validate historical findings using contemporary reproducibility frameworks?
- Replication studies : Follow protocols from Ferner & Runge (1955) but with larger cohorts and blinded analysis.
- Open-access data : Share raw datasets (e.g., blood glucose trajectories) in repositories like Figshare to enable meta-analyses .
Q. Methodological Best Practices
Q. What statistical tools are recommended for analyzing this compound’s heterogeneous data?
- Mixed-effects models : Account for variability in historical patient subgroups (e.g., age, BMI).
- Bayesian meta-analysis : Quantify uncertainty in small historical samples and infer dose-response relationships .
Q. How should researchers document this compound studies to ensure reproducibility?
- FAIR principles : Ensure data are Findable, Accessible, Interoperable, and Reusable.
- Pre-registration : Detail hypotheses and analysis plans on platforms like OSF to reduce bias .
Propriétés
IUPAC Name |
2-[10-(diaminomethylideneamino)decyl]guanidine | |
---|---|---|
Source | PubChem | |
URL | https://pubchem.ncbi.nlm.nih.gov | |
Description | Data deposited in or computed by PubChem | |
InChI |
InChI=1S/C12H28N6/c13-11(14)17-9-7-5-3-1-2-4-6-8-10-18-12(15)16/h1-10H2,(H4,13,14,17)(H4,15,16,18) | |
Source | PubChem | |
URL | https://pubchem.ncbi.nlm.nih.gov | |
Description | Data deposited in or computed by PubChem | |
InChI Key |
OZZMUVKANAPKGI-UHFFFAOYSA-N | |
Source | PubChem | |
URL | https://pubchem.ncbi.nlm.nih.gov | |
Description | Data deposited in or computed by PubChem | |
Canonical SMILES |
C(CCCCCN=C(N)N)CCCCN=C(N)N | |
Source | PubChem | |
URL | https://pubchem.ncbi.nlm.nih.gov | |
Description | Data deposited in or computed by PubChem | |
Molecular Formula |
C12H28N6 | |
Source | PubChem | |
URL | https://pubchem.ncbi.nlm.nih.gov | |
Description | Data deposited in or computed by PubChem | |
Related CAS |
301-15-5 (di-hydrochloride) | |
Record name | Synthalin A | |
Source | ChemIDplus | |
URL | https://pubchem.ncbi.nlm.nih.gov/substance/?source=chemidplus&sourceid=0000111239 | |
Description | ChemIDplus is a free, web search system that provides access to the structure and nomenclature authority files used for the identification of chemical substances cited in National Library of Medicine (NLM) databases, including the TOXNET system. | |
DSSTOX Substance ID |
DTXSID50911935 | |
Record name | N,N'-Decane-1,10-diyldiguanidine | |
Source | EPA DSSTox | |
URL | https://comptox.epa.gov/dashboard/DTXSID50911935 | |
Description | DSSTox provides a high quality public chemistry resource for supporting improved predictive toxicology. | |
Molecular Weight |
256.39 g/mol | |
Source | PubChem | |
URL | https://pubchem.ncbi.nlm.nih.gov | |
Description | Data deposited in or computed by PubChem | |
CAS No. |
111-23-9 | |
Record name | N,N′′′-1,10-Decanediylbis[guanidine] | |
Source | CAS Common Chemistry | |
URL | https://commonchemistry.cas.org/detail?cas_rn=111-23-9 | |
Description | CAS Common Chemistry is an open community resource for accessing chemical information. Nearly 500,000 chemical substances from CAS REGISTRY cover areas of community interest, including common and frequently regulated chemicals, and those relevant to high school and undergraduate chemistry classes. This chemical information, curated by our expert scientists, is provided in alignment with our mission as a division of the American Chemical Society. | |
Explanation | The data from CAS Common Chemistry is provided under a CC-BY-NC 4.0 license, unless otherwise stated. | |
Record name | Synthalin A | |
Source | ChemIDplus | |
URL | https://pubchem.ncbi.nlm.nih.gov/substance/?source=chemidplus&sourceid=0000111239 | |
Description | ChemIDplus is a free, web search system that provides access to the structure and nomenclature authority files used for the identification of chemical substances cited in National Library of Medicine (NLM) databases, including the TOXNET system. | |
Record name | N,N'-Decane-1,10-diyldiguanidine | |
Source | EPA DSSTox | |
URL | https://comptox.epa.gov/dashboard/DTXSID50911935 | |
Description | DSSTox provides a high quality public chemistry resource for supporting improved predictive toxicology. | |
Record name | N,N'''-1,10-decanediylbisguanidine | |
Source | European Chemicals Agency (ECHA) | |
URL | https://echa.europa.eu/substance-information/-/substanceinfo/100.003.499 | |
Description | The European Chemicals Agency (ECHA) is an agency of the European Union which is the driving force among regulatory authorities in implementing the EU's groundbreaking chemicals legislation for the benefit of human health and the environment as well as for innovation and competitiveness. | |
Explanation | Use of the information, documents and data from the ECHA website is subject to the terms and conditions of this Legal Notice, and subject to other binding limitations provided for under applicable law, the information, documents and data made available on the ECHA website may be reproduced, distributed and/or used, totally or in part, for non-commercial purposes provided that ECHA is acknowledged as the source: "Source: European Chemicals Agency, http://echa.europa.eu/". Such acknowledgement must be included in each copy of the material. ECHA permits and encourages organisations and individuals to create links to the ECHA website under the following cumulative conditions: Links can only be made to webpages that provide a link to the Legal Notice page. | |
Record name | 1,10-DIGUANIDINODECANE | |
Source | FDA Global Substance Registration System (GSRS) | |
URL | https://gsrs.ncats.nih.gov/ginas/app/beta/substances/ZEA6Y34XYT | |
Description | The FDA Global Substance Registration System (GSRS) enables the efficient and accurate exchange of information on what substances are in regulated products. Instead of relying on names, which vary across regulatory domains, countries, and regions, the GSRS knowledge base makes it possible for substances to be defined by standardized, scientific descriptions. | |
Explanation | Unless otherwise noted, the contents of the FDA website (www.fda.gov), both text and graphics, are not copyrighted. They are in the public domain and may be republished, reprinted and otherwise used freely by anyone without the need to obtain permission from FDA. Credit to the U.S. Food and Drug Administration as the source is appreciated but not required. | |
Retrosynthesis Analysis
AI-Powered Synthesis Planning: Our tool employs the Template_relevance Pistachio, Template_relevance Bkms_metabolic, Template_relevance Pistachio_ringbreaker, Template_relevance Reaxys, Template_relevance Reaxys_biocatalysis model, leveraging a vast database of chemical reactions to predict feasible synthetic routes.
One-Step Synthesis Focus: Specifically designed for one-step synthesis, it provides concise and direct routes for your target compounds, streamlining the synthesis process.
Accurate Predictions: Utilizing the extensive PISTACHIO, BKMS_METABOLIC, PISTACHIO_RINGBREAKER, REAXYS, REAXYS_BIOCATALYSIS database, our tool offers high-accuracy predictions, reflecting the latest in chemical research and data.
Strategy Settings
Precursor scoring | Relevance Heuristic |
---|---|
Min. plausibility | 0.01 |
Model | Template_relevance |
Template Set | Pistachio/Bkms_metabolic/Pistachio_ringbreaker/Reaxys/Reaxys_biocatalysis |
Top-N result to add to graph | 6 |
Feasible Synthetic Routes
Avertissement et informations sur les produits de recherche in vitro
Veuillez noter que tous les articles et informations sur les produits présentés sur BenchChem sont destinés uniquement à des fins informatives. Les produits disponibles à l'achat sur BenchChem sont spécifiquement conçus pour des études in vitro, qui sont réalisées en dehors des organismes vivants. Les études in vitro, dérivées du terme latin "in verre", impliquent des expériences réalisées dans des environnements de laboratoire contrôlés à l'aide de cellules ou de tissus. Il est important de noter que ces produits ne sont pas classés comme médicaments et n'ont pas reçu l'approbation de la FDA pour la prévention, le traitement ou la guérison de toute condition médicale, affection ou maladie. Nous devons souligner que toute forme d'introduction corporelle de ces produits chez les humains ou les animaux est strictement interdite par la loi. Il est essentiel de respecter ces directives pour assurer la conformité aux normes légales et éthiques en matière de recherche et d'expérimentation.