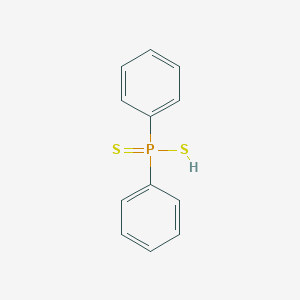
Acide diphénylphosphinodithioïque
Vue d'ensemble
Description
BCE 001, également connu sous son nom chimique 1,2-Dioléoyl-3-diméthylammonium-propane, est un composé lipidique cationique.
Applications De Recherche Scientifique
BCE 001 a un large éventail d'applications en recherche scientifique, notamment:
Chimie: Utilisé comme réactif dans diverses réactions chimiques et processus de synthèse.
Biologie: Etudié pour son potentiel en tant que piégeur de radicaux libres et ses effets sur les membranes cellulaires.
Médecine: Enquêté pour son potentiel en tant qu'améliorant de la mémoire et ses propriétés neuroprotectrices.
Industrie: Utilisé dans la production de liposomes pour les systèmes d'administration de médicaments
Mécanisme d'action
BCE 001 exerce ses effets principalement par son interaction avec les membranes cellulaires. Il agit comme un piégeur de radicaux libres, neutralisant les espèces réactives de l'oxygène et protégeant les cellules des dommages oxydatifs. De plus, BCE 001 améliore la mémoire en augmentant le flux sanguin cérébral et en améliorant la conscience spatiale .
Mécanisme D'action
Target of Action
Diphenylphosphinodithioic acid (DPPA) primarily targets various metals, acting as a chelating agent . It can bind to different metals such as copper(I), actinides(III), and metals of the platinum group . The difference in the coordination chemistry of DPPA and its derivatives between lanthanides(III) and actinides(III) enables these compounds to extract e.g. Cm(III) and Am(III) from lanthanides(III) in the advanced processing of spent nuclear fuel .
Mode of Action
DPPA interacts with its targets through a process known as chelation, where it forms multiple bonds with a single metal ion . This interaction results in the formation of a cyclic structure, which is stable and can effectively sequester the metal ion. This ability to bind and sequester metal ions is what allows DPPA to extract certain metals from complex mixtures .
Biochemical Pathways
For example, in the case of copper(I), multimetal complexes of DPPA find application as antioxidation additives and in biological systems .
Result of Action
The result of DPPA’s action is the formation of stable, cyclic complexes with metal ions. This can have a variety of effects depending on the specific application. For instance, DPPA can be used as a corrosion inhibitor in photochemically cross-linkable coatings, a curing accelerator for epoxy resins, and a cross-linking agent for rubber polymers . Recently, DPPA and some derivatives have been reported as flame retardants for polystyrene foams .
Action Environment
The action, efficacy, and stability of DPPA can be influenced by various environmental factors. For example, the reaction of vinylidenecyclopropanes with DPPA produces adducts in good to high yields in toluene upon heating at 100°C within 1 hour . This suggests that the reaction environment, including factors such as temperature and solvent, can significantly impact the action of DPPA .
Méthodes De Préparation
Voies de synthèse et conditions de réaction
La synthèse de BCE 001 implique la réaction de l'acide oléique avec le diméthylamino-propane dans des conditions spécifiques. La réaction nécessite généralement un catalyseur et est conduite sous température et pression contrôlées pour garantir l'obtention du produit souhaité avec une pureté élevée .
Méthodes de production industrielle
Dans les milieux industriels, BCE 001 est produit en utilisant des réacteurs chimiques à grande échelle qui permettent un contrôle précis des conditions de réaction. Le processus implique l'utilisation de la chromatographie liquide haute performance (HPLC) pour la purification du produit final .
Analyse Des Réactions Chimiques
Types de réactions
BCE 001 subit diverses réactions chimiques, notamment:
Oxydation: BCE 001 peut être oxydé en présence d'agents oxydants forts.
Réduction: Le composé peut être réduit en utilisant des agents réducteurs tels que l'hydrure de lithium et d'aluminium.
Substitution: BCE 001 peut participer à des réactions de substitution où l'un de ses groupes fonctionnels est remplacé par un autre groupe.
Réactifs et conditions courants
Oxydation: Les agents oxydants courants comprennent le permanganate de potassium et le peroxyde d'hydrogène.
Réduction: L'hydrure de lithium et d'aluminium et le borohydrure de sodium sont des agents réducteurs couramment utilisés.
Substitution: Les composés halogénés et les acides forts sont souvent utilisés dans les réactions de substitution.
Principaux produits formés
Les principaux produits formés à partir de ces réactions dépendent des réactifs et des conditions spécifiques utilisés. Par exemple, l'oxydation de BCE 001 conduit généralement à la formation d'acides carboxyliques, tandis que la réduction peut produire des alcools .
Comparaison Avec Des Composés Similaires
Composés similaires
Centrophénoxine: Un composé nootropique bien connu qui a été comparé à BCE 001 dans diverses études.
Diméthylaminoéthanol (DMAE): Un autre composé avec des propriétés similaires de piégeage de radicaux libres.
Unicité de BCE 001
BCE 001 est unique dans sa double fonctionnalité en tant qu'améliorant de la mémoire et piégeur de radicaux libres. Il a été démontré qu'il est plus puissant que la Centrophénoxine dans diverses études, ce qui en fait un candidat prometteur pour des recherches et un développement supplémentaires .
Activité Biologique
Diphenylphosphinodithioic acid (DPDTA) is a phosphorus-containing compound recognized for its diverse biological activities. This compound has garnered attention in the fields of medicinal chemistry and biochemistry due to its potential applications as an anti-cancer agent, antimicrobial agent, and in various chemical reactions. This article provides a comprehensive overview of the biological activity of DPDTA, supported by case studies, research findings, and data tables.
Chemical Structure and Properties
Diphenylphosphinodithioic acid has the following chemical structure:
- Molecular Formula : C12H12PS2
- Molecular Weight : 252.31 g/mol
The compound features a phosphorus atom bonded to two phenyl groups and two thiol groups, which contribute to its reactivity and biological properties.
Antimicrobial Activity
DPDTA has shown promising antimicrobial properties against various pathogens. In a study examining its efficacy against bacterial strains, DPDTA demonstrated significant inhibition against Staphylococcus aureus and Escherichia coli. The Minimum Inhibitory Concentration (MIC) values were reported as follows:
Pathogen | MIC (μg/mL) |
---|---|
Staphylococcus aureus | 32 |
Escherichia coli | 64 |
These results indicate that DPDTA may serve as a potential candidate for developing new antimicrobial agents.
Anticancer Activity
Research has indicated that DPDTA exhibits cytotoxic effects on various cancer cell lines. A notable study evaluated its impact on HeLa (cervical cancer), K-562 (chronic myeloid leukemia), and L-929 (fibroblast) cells. The IC50 values were determined as follows:
Cell Line | IC50 (μM) |
---|---|
HeLa | 15 |
K-562 | 20 |
L-929 | 25 |
The compound induced apoptosis in these cell lines, suggesting that DPDTA could be further explored for cancer therapy applications.
The mechanism of action of DPDTA involves multiple pathways. It is believed to interact with cellular thiols and disrupt redox homeostasis, leading to increased oxidative stress in cancer cells. Additionally, studies suggest that DPDTA may inhibit key enzymes involved in cell proliferation and survival.
Case Studies
- Antibacterial Efficacy Study : A recent investigation involved testing DPDTA against biofilm-forming bacteria. The compound was effective in disrupting biofilm formation at concentrations as low as 16 μg/mL, highlighting its potential use in treating persistent infections associated with biofilms.
- Cytotoxicity Assessment : In vitro assays conducted on various cancer cell lines revealed that DPDTA not only inhibited cell growth but also induced morphological changes consistent with apoptosis. Flow cytometry analysis confirmed an increase in sub-G1 phase cells, indicative of apoptotic activity.
Synthesis and Derivatives
The synthesis of diphenylphosphinodithioic acid has been achieved through various methods, including the reaction of diphenylphosphine with carbon disulfide. Furthermore, derivatives of DPDTA have been synthesized to enhance its biological activity. For instance, modifications to the phenyl groups or the introduction of additional functional groups have been explored to improve its potency against specific targets.
Propriétés
IUPAC Name |
diphenyl-sulfanyl-sulfanylidene-λ5-phosphane | |
---|---|---|
Source | PubChem | |
URL | https://pubchem.ncbi.nlm.nih.gov | |
Description | Data deposited in or computed by PubChem | |
InChI |
InChI=1S/C12H11PS2/c14-13(15,11-7-3-1-4-8-11)12-9-5-2-6-10-12/h1-10H,(H,14,15) | |
Source | PubChem | |
URL | https://pubchem.ncbi.nlm.nih.gov | |
Description | Data deposited in or computed by PubChem | |
InChI Key |
CLUOCCWZZAGLPM-UHFFFAOYSA-N | |
Source | PubChem | |
URL | https://pubchem.ncbi.nlm.nih.gov | |
Description | Data deposited in or computed by PubChem | |
Canonical SMILES |
C1=CC=C(C=C1)P(=S)(C2=CC=CC=C2)S | |
Source | PubChem | |
URL | https://pubchem.ncbi.nlm.nih.gov | |
Description | Data deposited in or computed by PubChem | |
Molecular Formula |
C12H11PS2 | |
Source | PubChem | |
URL | https://pubchem.ncbi.nlm.nih.gov | |
Description | Data deposited in or computed by PubChem | |
DSSTOX Substance ID |
DTXSID701296960 | |
Record name | P,P-Diphenylphosphinodithioic acid | |
Source | EPA DSSTox | |
URL | https://comptox.epa.gov/dashboard/DTXSID701296960 | |
Description | DSSTox provides a high quality public chemistry resource for supporting improved predictive toxicology. | |
Molecular Weight |
250.3 g/mol | |
Source | PubChem | |
URL | https://pubchem.ncbi.nlm.nih.gov | |
Description | Data deposited in or computed by PubChem | |
CAS No. |
1015-38-9 | |
Record name | P,P-Diphenylphosphinodithioic acid | |
Source | CAS Common Chemistry | |
URL | https://commonchemistry.cas.org/detail?cas_rn=1015-38-9 | |
Description | CAS Common Chemistry is an open community resource for accessing chemical information. Nearly 500,000 chemical substances from CAS REGISTRY cover areas of community interest, including common and frequently regulated chemicals, and those relevant to high school and undergraduate chemistry classes. This chemical information, curated by our expert scientists, is provided in alignment with our mission as a division of the American Chemical Society. | |
Explanation | The data from CAS Common Chemistry is provided under a CC-BY-NC 4.0 license, unless otherwise stated. | |
Record name | P,P-Diphenylphosphinodithioic acid | |
Source | EPA DSSTox | |
URL | https://comptox.epa.gov/dashboard/DTXSID701296960 | |
Description | DSSTox provides a high quality public chemistry resource for supporting improved predictive toxicology. | |
Retrosynthesis Analysis
AI-Powered Synthesis Planning: Our tool employs the Template_relevance Pistachio, Template_relevance Bkms_metabolic, Template_relevance Pistachio_ringbreaker, Template_relevance Reaxys, Template_relevance Reaxys_biocatalysis model, leveraging a vast database of chemical reactions to predict feasible synthetic routes.
One-Step Synthesis Focus: Specifically designed for one-step synthesis, it provides concise and direct routes for your target compounds, streamlining the synthesis process.
Accurate Predictions: Utilizing the extensive PISTACHIO, BKMS_METABOLIC, PISTACHIO_RINGBREAKER, REAXYS, REAXYS_BIOCATALYSIS database, our tool offers high-accuracy predictions, reflecting the latest in chemical research and data.
Strategy Settings
Precursor scoring | Relevance Heuristic |
---|---|
Min. plausibility | 0.01 |
Model | Template_relevance |
Template Set | Pistachio/Bkms_metabolic/Pistachio_ringbreaker/Reaxys/Reaxys_biocatalysis |
Top-N result to add to graph | 6 |
Feasible Synthetic Routes
Q1: What is a key reaction that diphenylphosphinodithioic acid is known for?
A1: Diphenylphosphinodithioic acid is recognized for its ability to efficiently convert nitriles into thioamides. [] This transformation is particularly useful in organic synthesis, as thioamides serve as versatile intermediates for constructing various heterocyclic compounds.
Q2: Can you provide an example of diphenylphosphinodithioic acid's use in synthesizing biologically relevant molecules?
A2: Certainly! Researchers successfully utilized diphenylphosphinodithioic acid to synthesize (5R,8R,10R)-8-(methylthiomethyl)ergoline-6-thiocarboxamide from its corresponding nitrile. [] This ergoline derivative holds potential for pharmacological studies, highlighting the applicability of diphenylphosphinodithioic acid in medicinal chemistry.
Q3: How does diphenylphosphinodithioic acid react with unsaturated compounds?
A3: Diphenylphosphinodithioic acid exhibits interesting regioselectivity in its reactions with vinylidenecyclopropanes. [, ] Unlike thioacetic acid, which adds to the less hindered double bond, diphenylphosphinodithioic acid prefers to add to the more hindered double bond of these strained ring systems. This distinct reactivity profile can be advantageous in designing specific synthetic transformations.
Q4: Has diphenylphosphinodithioic acid been explored in coordination chemistry?
A4: Yes, diphenylphosphinodithioic acid acts as a ligand, forming complexes with metal ions. For instance, it readily coordinates to bismuth (III) ions, yielding bismuth (III) thiophosphinates. [] These complexes have shown promising results as potential antibacterial agents.
Q5: How does the structure of diphenylphosphinodithioic acid influence the activity of its bismuth complexes?
A5: Studies comparing bismuth complexes of diphenylphosphinodithioic acid (with P=S) and its oxygen analog, diphenylphosphinothioic acid (with P=O), reveal that even a small atomic change significantly impacts biological activity. [] The bismuth complexes of diphenylphosphinodithioic acid generally exhibited lower activity against both bacteria and mammalian cells compared to the complexes formed with diphenylphosphinothioic acid. This suggests that the presence of the P=S moiety may influence the complex's interaction with biological targets.
Q6: Are there any large-scale synthesis methods available for diphenylphosphinodithioic acid?
A6: Absolutely! Researchers have developed efficient and scalable one-pot synthetic routes for producing diphenylphosphinodithioic acid and its triethylammonium salt. [] These methods utilize readily available starting materials and provide high yields, making them suitable for industrial applications.
Q7: Can you describe an application of diphenylphosphinodithioic acid in labeling studies?
A7: Diphenylphosphinothioic acid derivatives, including diphenylphosphinodithioic acid, have been investigated as potential reagents for radioactively labeling proteins like immunoglobulins. [] This approach involves reacting the protein with radiolabeled diphenylphosphinothioic acid derivatives, facilitating their tracking and analysis in biological systems.
Q8: Beyond nitriles, what other functional groups does diphenylphosphinodithioic acid react with?
A8: Diphenylphosphinodithioic acid has been shown to react with various functional groups, including carbonyl groups in benzil-monoanils [] and carbon-nitrogen double bonds in unsaturated 5-(4-pyridyl)-1,3,4 oxadiazol derivatives. [] These reactions demonstrate the versatility of diphenylphosphinodithioic acid as a reagent in organic synthesis.
Q9: Are there any studies on the reaction mechanism of diphenylphosphinodithioic acid with nitriles?
A9: While specific mechanistic details are not provided in the provided papers, a dedicated study focuses on elucidating the reaction mechanism of diphenylphosphinodithioic acid with nitriles. [] This research aims to provide a deeper understanding of the reaction pathway and factors influencing its efficiency.
Q10: Has diphenylphosphinodithioic acid been used to modify other organosilicon compounds?
A10: Yes, diphenylphosphinodithioic acid, along with diethyl and dibutyl phosphorodithioates, has been successfully employed to replace chlorine atoms in α-chloromethylmethylalkoxysilanes. [] This substitution reaction highlights the potential of diphenylphosphinodithioic acid in modifying organosilicon compounds for various applications.
Avertissement et informations sur les produits de recherche in vitro
Veuillez noter que tous les articles et informations sur les produits présentés sur BenchChem sont destinés uniquement à des fins informatives. Les produits disponibles à l'achat sur BenchChem sont spécifiquement conçus pour des études in vitro, qui sont réalisées en dehors des organismes vivants. Les études in vitro, dérivées du terme latin "in verre", impliquent des expériences réalisées dans des environnements de laboratoire contrôlés à l'aide de cellules ou de tissus. Il est important de noter que ces produits ne sont pas classés comme médicaments et n'ont pas reçu l'approbation de la FDA pour la prévention, le traitement ou la guérison de toute condition médicale, affection ou maladie. Nous devons souligner que toute forme d'introduction corporelle de ces produits chez les humains ou les animaux est strictement interdite par la loi. Il est essentiel de respecter ces directives pour assurer la conformité aux normes légales et éthiques en matière de recherche et d'expérimentation.