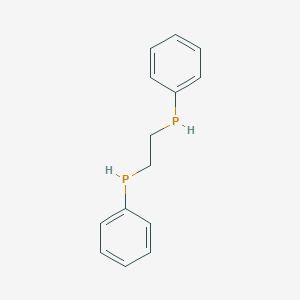
1,2-Bis(phenylphosphino)ethane
Vue d'ensemble
Description
1,2-Bis(phenylphosphino)ethane (CAS: 1663-45-2), commonly abbreviated as DPPE in literature, is a bidentate diphosphine ligand with the molecular formula C₂₆H₂₄P₂ and a molecular weight of 398.42 g/mol. It features two phenylphosphine groups linked by an ethylene backbone. Key properties include a melting point of 138–142°C, high purity (≥98%), and applications as an intermediate in organic synthesis and coordination chemistry . DPPE is synthesized via established methods involving reactions of phenylphosphine precursors with ethylene derivatives . Its rigid structure and strong electron-donating capacity make it a versatile ligand in catalysis, antitumor agents, and materials science .
Méthodes De Préparation
The preparation of 1,2-Bis(phenylphosphino)ethane typically involves the alkylation of sodium diphenylphosphide with 1,2-dichloroethane. The reaction proceeds as follows :
[ 2 NaP(C_6H_5)_2 + ClCH_2CH_2Cl \rightarrow (C_6H_5)_2PCH_2CH_2P(C_6H_5)_2 + 2 NaCl ]
This method is commonly used in laboratory settings. Industrial production methods may involve similar synthetic routes but on a larger scale, with optimizations for yield and purity.
Analyse Des Réactions Chimiques
1,2-Bis(phenylphosphino)ethane undergoes various chemical reactions, including:
-
Reduction: : The reduction of this compound by lithium yields the corresponding secondary phosphine .
[ (C_6H_5)_2PCH_2CH_2P(C_6H_5)_2 + 4 Li \rightarrow Li(C_6H_5)PCH_2CH_2P(C_6H_5)Li + 2 C_6H_5Li ]
-
Oxidation: : Treatment with hydrogen peroxide produces phosphine oxides .
[ (C_6H_5)_2PCH_2CH_2P(C_6H_5)_2 + H_2O_2 \rightarrow (C_6H_5)_2P(O)CH_2CH_2P(C_6H_5)_2 ]
-
Substitution: : The compound can undergo substitution reactions with various reagents to form different derivatives .
Applications De Recherche Scientifique
1,2-Bis(phenylphosphino)ethane is widely used in scientific research due to its ability to form stable metal complexes. Some of its applications include:
Mécanisme D'action
The mechanism by which 1,2-Bis(phenylphosphino)ethane exerts its effects is primarily through chelation. The bidentate ligand forms a stable five-membered ring with metal centers, providing an entropic advantage over monodentate ligands. This chelation effect stabilizes the metal complex, making it more effective in catalytic processes .
Comparaison Avec Des Composés Similaires
Comparison with Structurally Similar Compounds
1,2-Bis(dimethylphosphino)ethane (DMPE)
- Structure : Methyl groups replace phenyl substituents, yielding a molecular formula C₆H₁₆P₂ and molecular weight 150.14 g/mol .
- Synthesis : Prepared via NaH/LiAlH₄ activation and reduction of phosphorylated intermediates .
- Applications : Used in coordination chemistry for smaller, less sterically hindered complexes.
- Key Differences :
1,2-Bis(diphenylarsino)ethane
- Structure : Arsenic replaces phosphorus atoms, forming a heavier analog.
- Applications : Explored in quantum information processing for altered decoherence properties.
- Key Differences: Arsenic’s larger atomic radius and weaker donor strength reduce ligand field stabilization compared to DPPE. Demonstrated unique spin dynamics in para-hydrogen-enhanced NMR studies .
(R,R)-1,2-Bis[(2-methoxyphenyl)(phenylphosphino)]ethane (DIPAMP)
- Structure : Chiral methoxyphenyl groups introduce enantioselectivity.
- Applications :
- Key Differences :
(R,R)-1,2-Bis(tert-butylphenylphosphino)ethane (BisP*)
- Structure : tert-Butylphenyl groups provide extreme steric bulk.
- Applications: Rh-catalyzed asymmetric hydrogenation of α-dehydroamino acids.
- Key Differences: Outperforms DIPAMP in enantioselectivity (e.g., >99% ee in hydrogenation reactions) due to rigid, bulky substituents . Limited solubility in non-polar solvents compared to DPPE.
1,2-Bis(diethylphosphino)ethane
- Structure : Ethyl groups replace phenyl substituents.
- Applications : Molecular electronics, particularly in single-molecule junctions.
- Key Differences: Intermediate steric bulk between DMPE and DPPE. Used in Fe and Mo complexes for studying charge transport in nanoscale devices .
Comparative Data Table
Antitumor Activity
- DPPE’s gold(I) complex, [Au(DPPE)₂]Cl, exhibits 87% increased lifespan in murine P388 leukemia models. It induces DNA-protein crosslinks and inhibits protein synthesis preferentially .
- Mechanism : Stability in serum and resistance to thiol displacement contribute to its efficacy, unlike other Au(I) complexes .
Asymmetric Catalysis
- DIPAMP achieves >95% enantiomeric excess in hydrogenation of methyl-(Z)-α-acetamidocinnamate. Solvent viscosity impacts H₂ mass transfer, affecting reaction rates .
- BisP* surpasses DIPAMP in enantioselectivity, attributed to its rigid tert-butyl groups .
Materials Science
- DPPE forms mixed-ligand Au₁₃ clusters with DIPAMP, displaying tunable optical activity. Reverse-phase HPLC isolates clusters with x = 1–5 DIPAMP ligands, all showing CD/CPL signals .
- In molecular junctions, DPPE’Mo complexes exhibit bias-dependent conductance switching, relevant to nanoelectronics .
Activité Biologique
1,2-Bis(phenylphosphino)ethane (DPPE) is a bidentate phosphine ligand extensively utilized in coordination chemistry and catalysis. Its biological activity has garnered attention, particularly in the context of anticancer agents and metal complexes. This article reviews the biological activity of DPPE and its metal complexes, highlighting their mechanisms of action, cytotoxicity profiles, and potential therapeutic applications.
Chemical Structure and Properties
DPPE features a central ethane backbone with two phenylphosphino groups attached. This configuration allows for chelation with various transition metals, enhancing the stability and reactivity of the resulting complexes.
Anticancer Properties
Recent studies have demonstrated that gold(I) and copper(I) complexes of DPPE exhibit significant anticancer activity. Notably, these complexes trigger apoptosis in cancer cells through various mechanisms:
- Gold(I)-Phosphine Complexes : These complexes have shown potent antiproliferative effects against multiple cancer cell lines. They induce apoptosis by targeting mitochondrial functions and inhibiting thioredoxin reductase, leading to increased reactive oxygen species (ROS) production .
- Copper(I)-DPPE Complexes : Research indicates that copper(I) complexes of DPPE also exhibit cytotoxicity against human cancer cell lines. The mechanism involves ROS generation and disruption of cellular redox homeostasis, contributing to cell death .
Mechanistic Insights
The biological activity of DPPE and its metal complexes is largely attributed to their ability to form stable coordination compounds that can interact with biological macromolecules. The following mechanisms have been proposed:
- Mitochondrial Dysfunction : Both gold and copper complexes disrupt mitochondrial function, leading to increased ROS levels that trigger apoptotic pathways .
- Inhibition of Key Enzymes : The inhibition of thioredoxin reductase by gold complexes impairs cellular antioxidant defenses, making cancer cells more susceptible to oxidative stress .
Study 1: Gold(I)-DPPE Complexes
A study investigated the in vitro cytotoxicity of bis[1,2-bis(diphenylphosphino)ethane]gold(I) chloride against ovarian cancer cells. The results indicated a significant reduction in cell viability and increased apoptosis markers compared to untreated controls. The study emphasized the importance of the phosphine ligand in enhancing the anticancer properties of gold complexes .
Study 2: Copper(I)-DPPE Complexes
Research on copper(I) complexes revealed their potential as therapeutic agents against human cancer cells. The study highlighted the cytotoxic effects observed at low concentrations, suggesting that these complexes could serve as lead compounds for further drug development .
Q & A
Basic Research Questions
Q. What are the common synthetic routes for 1,2-Bis(phenylphosphino)ethane, and what parameters are critical for optimizing yield?
- Methodological Answer : The synthesis typically involves reacting phenylphosphine derivatives with ethylene-based precursors under inert conditions. A reported method starts with this compound reacting with formaldehyde to form cyclic aminomethylphosphines, requiring strict control of stoichiometry (1:2 molar ratio) and temperature (60–80°C) . Yield optimization depends on maintaining anhydrous conditions (e.g., using Schlenk techniques) and purifying intermediates via recrystallization from toluene/hexane mixtures. Air-sensitive intermediates necessitate nitrogen atmospheres during handling .
Q. How should this compound be handled and stored to prevent degradation?
- Methodological Answer : Due to its air sensitivity and flammability (GHS Class 3), the compound must be stored under nitrogen or argon in flame-resistant containers at temperatures below 25°C . Handling requires gloveboxes or Schlenk lines to avoid oxidation. Immediate post-synthesis purification (e.g., column chromatography under nitrogen) minimizes decomposition. Safety protocols include using flame-resistant labware and avoiding contact with oxidizing agents .
Q. What spectroscopic and analytical techniques are effective for characterizing purity and structure?
- Methodological Answer :
- ESI Mass Spectrometry : Confirms molecular weight (e.g., [M+H]+ peak at m/z 399.4) .
- Melting Point Analysis : Pure samples melt sharply at 138–142°C .
- X-ray Crystallography : Resolves bond lengths (e.g., P–C = 1.81 Å) and conformational details (e.g., gauche P–P arrangement) .
- 31P NMR : Detects impurities via distinct phosphorus environments (δ ~ –15 ppm for free ligand) .
Advanced Research Questions
Q. How does the coordination geometry of this compound influence its efficacy in transition metal catalysis?
- Methodological Answer : The ligand’s bidentate P^P chelation stabilizes square-planar (e.g., Rh(I)) or octahedral (e.g., Mo(IV)) metal centers, enhancing catalytic activity. In Rh-catalyzed asymmetric hydrogenation, the bite angle (~85°) and electron-donating phenyl groups modulate substrate binding and enantioselectivity. Comparative studies with DIPAMP (a chiral analog) show differences in steric bulk and π-backbonding efficiency .
Q. How can solvent effects resolve contradictions in reported catalytic activities of metal complexes using this ligand?
- Methodological Answer : Discrepancies in catalytic efficiency (e.g., turnover frequency) often arise from solvent polarity and viscosity. For example, high-viscosity solvents (e.g., glycerol) reduce gas diffusion rates in hydrogenation, lowering activity. Systematic solvent screening (e.g., using Kamlet-Taft parameters) and kinetic profiling under controlled conditions (e.g., CO₂-expanded liquids) can isolate solvent-specific effects .
Q. What mechanistic insights explain the role of solvent choice in Rhodium-catalyzed asymmetric hydrogenation using this ligand?
- Methodological Answer : Solvent polarity affects transition-state stabilization. Polar aprotic solvents (e.g., DMF) enhance substrate-ligand interactions via dipole-dipole forces, improving enantiomeric excess (ee). Conversely, low-polarity solvents (e.g., toluene) favor π-π interactions between phenyl groups and aromatic substrates. Solvent viscosity also impacts mass transfer; gas-sparging reactors mitigate H₂ diffusion limitations .
Q. How are X-ray crystallography and SHELXL refinement used to analyze structural conformations of oxidized derivatives?
- Methodological Answer : Single-crystal X-ray diffraction (e.g., Mo Kα radiation, λ = 0.71073 Å) resolves oxidation products like meso-PhHPO–CH₂–CH₂–OPHPh. SHELXL refines anisotropic displacement parameters and hydrogen positions via riding models (Uiso(H) = 1.2Ueq(C/P)). Conformational analysis (e.g., P–O bond alignment with phenyl rings) reveals steric strain and hydrogen-bonding networks (C–H⋯O interactions) .
Q. How does this compound compare to other diphosphine ligands in stabilizing metal complexes?
- Methodological Answer : Comparative studies using cyclic voltammetry and DFT calculations show that its smaller bite angle vs. 1,3-Bis(diphenylphosphino)propane (dppp) increases metal-ligand bond strength. However, bulkier ligands like BINAP provide better steric shielding for chiral centers. Catalytic performance in cross-coupling (e.g., Suzuki-Miyaura) correlates with ligand flexibility and electron density .
Propriétés
IUPAC Name |
phenyl(2-phenylphosphanylethyl)phosphane | |
---|---|---|
Source | PubChem | |
URL | https://pubchem.ncbi.nlm.nih.gov | |
Description | Data deposited in or computed by PubChem | |
InChI |
InChI=1S/C14H16P2/c1-3-7-13(8-4-1)15-11-12-16-14-9-5-2-6-10-14/h1-10,15-16H,11-12H2 | |
Source | PubChem | |
URL | https://pubchem.ncbi.nlm.nih.gov | |
Description | Data deposited in or computed by PubChem | |
InChI Key |
VBXSERPGINMTDE-UHFFFAOYSA-N | |
Source | PubChem | |
URL | https://pubchem.ncbi.nlm.nih.gov | |
Description | Data deposited in or computed by PubChem | |
Canonical SMILES |
C1=CC=C(C=C1)PCCPC2=CC=CC=C2 | |
Source | PubChem | |
URL | https://pubchem.ncbi.nlm.nih.gov | |
Description | Data deposited in or computed by PubChem | |
Molecular Formula |
C14H16P2 | |
Source | PubChem | |
URL | https://pubchem.ncbi.nlm.nih.gov | |
Description | Data deposited in or computed by PubChem | |
DSSTOX Substance ID |
DTXSID00172276 | |
Record name | Ethylenebis(phenylphosphine) | |
Source | EPA DSSTox | |
URL | https://comptox.epa.gov/dashboard/DTXSID00172276 | |
Description | DSSTox provides a high quality public chemistry resource for supporting improved predictive toxicology. | |
Molecular Weight |
246.22 g/mol | |
Source | PubChem | |
URL | https://pubchem.ncbi.nlm.nih.gov | |
Description | Data deposited in or computed by PubChem | |
CAS No. |
18899-64-4 | |
Record name | 1,2-Bis(phenylphosphino)ethane | |
Source | CAS Common Chemistry | |
URL | https://commonchemistry.cas.org/detail?cas_rn=18899-64-4 | |
Description | CAS Common Chemistry is an open community resource for accessing chemical information. Nearly 500,000 chemical substances from CAS REGISTRY cover areas of community interest, including common and frequently regulated chemicals, and those relevant to high school and undergraduate chemistry classes. This chemical information, curated by our expert scientists, is provided in alignment with our mission as a division of the American Chemical Society. | |
Explanation | The data from CAS Common Chemistry is provided under a CC-BY-NC 4.0 license, unless otherwise stated. | |
Record name | Ethylenebis(phenylphosphine) | |
Source | ChemIDplus | |
URL | https://pubchem.ncbi.nlm.nih.gov/substance/?source=chemidplus&sourceid=0018899644 | |
Description | ChemIDplus is a free, web search system that provides access to the structure and nomenclature authority files used for the identification of chemical substances cited in National Library of Medicine (NLM) databases, including the TOXNET system. | |
Record name | Ethylenebis(phenylphosphine) | |
Source | EPA DSSTox | |
URL | https://comptox.epa.gov/dashboard/DTXSID00172276 | |
Description | DSSTox provides a high quality public chemistry resource for supporting improved predictive toxicology. | |
Record name | Ethylenebis[phenylphosphine] | |
Source | European Chemicals Agency (ECHA) | |
URL | https://echa.europa.eu/substance-information/-/substanceinfo/100.038.757 | |
Description | The European Chemicals Agency (ECHA) is an agency of the European Union which is the driving force among regulatory authorities in implementing the EU's groundbreaking chemicals legislation for the benefit of human health and the environment as well as for innovation and competitiveness. | |
Explanation | Use of the information, documents and data from the ECHA website is subject to the terms and conditions of this Legal Notice, and subject to other binding limitations provided for under applicable law, the information, documents and data made available on the ECHA website may be reproduced, distributed and/or used, totally or in part, for non-commercial purposes provided that ECHA is acknowledged as the source: "Source: European Chemicals Agency, http://echa.europa.eu/". Such acknowledgement must be included in each copy of the material. ECHA permits and encourages organisations and individuals to create links to the ECHA website under the following cumulative conditions: Links can only be made to webpages that provide a link to the Legal Notice page. | |
Retrosynthesis Analysis
AI-Powered Synthesis Planning: Our tool employs the Template_relevance Pistachio, Template_relevance Bkms_metabolic, Template_relevance Pistachio_ringbreaker, Template_relevance Reaxys, Template_relevance Reaxys_biocatalysis model, leveraging a vast database of chemical reactions to predict feasible synthetic routes.
One-Step Synthesis Focus: Specifically designed for one-step synthesis, it provides concise and direct routes for your target compounds, streamlining the synthesis process.
Accurate Predictions: Utilizing the extensive PISTACHIO, BKMS_METABOLIC, PISTACHIO_RINGBREAKER, REAXYS, REAXYS_BIOCATALYSIS database, our tool offers high-accuracy predictions, reflecting the latest in chemical research and data.
Strategy Settings
Precursor scoring | Relevance Heuristic |
---|---|
Min. plausibility | 0.01 |
Model | Template_relevance |
Template Set | Pistachio/Bkms_metabolic/Pistachio_ringbreaker/Reaxys/Reaxys_biocatalysis |
Top-N result to add to graph | 6 |
Feasible Synthetic Routes
Avertissement et informations sur les produits de recherche in vitro
Veuillez noter que tous les articles et informations sur les produits présentés sur BenchChem sont destinés uniquement à des fins informatives. Les produits disponibles à l'achat sur BenchChem sont spécifiquement conçus pour des études in vitro, qui sont réalisées en dehors des organismes vivants. Les études in vitro, dérivées du terme latin "in verre", impliquent des expériences réalisées dans des environnements de laboratoire contrôlés à l'aide de cellules ou de tissus. Il est important de noter que ces produits ne sont pas classés comme médicaments et n'ont pas reçu l'approbation de la FDA pour la prévention, le traitement ou la guérison de toute condition médicale, affection ou maladie. Nous devons souligner que toute forme d'introduction corporelle de ces produits chez les humains ou les animaux est strictement interdite par la loi. Il est essentiel de respecter ces directives pour assurer la conformité aux normes légales et éthiques en matière de recherche et d'expérimentation.