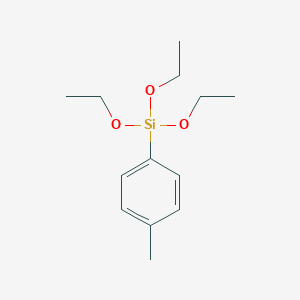
Triethoxy-p-tolylsilane
Vue d'ensemble
Description
L'érythrosphingosine, également connue sous le nom de D-érythro-sphingosine, est une base sphingoïde naturelle qui joue un rôle crucial dans la structure et la fonction des sphingolipides. Les sphingolipides sont des composants essentiels des membranes cellulaires et sont impliqués dans divers processus cellulaires, notamment la signalisation cellulaire, la prolifération et l'apoptose. L'érythrosphingosine se caractérise par sa structure d'alcool aminé aliphatique à longue chaîne, qui est essentielle à ses activités biologiques .
Mécanisme D'action
Triethoxy-p-tolylsilane, also known as Triethoxy(p-tolyl)silane, is an organosilicon compound with diverse applications in the chemical industry . It consists of a p-tolyl group, an oxygen atom, and three ethoxy groups . Here is a detailed analysis of its mechanism of action:
Pharmacokinetics
Organosilicon compounds are generally known for their stability and resistance to metabolism, which can impact their bioavailability .
Action Environment
The action, efficacy, and stability of this compound can be influenced by various environmental factors. For instance, it is known to react slowly with moisture/water . Therefore, the presence of water can potentially affect its stability and efficacy.
Méthodes De Préparation
Voies Synthétiques et Conditions de Réaction : L'érythrosphingosine peut être synthétisée par plusieurs méthodes. Une approche courante implique l'utilisation de matières premières chirales, telles que les glucides et les acides aminés, pour assurer la bonne stéréochimie. Par exemple, la D-ribo-phytosphingosine peut être utilisée comme matière première, qui subit une transformation sélective pour former l'érythrosphingosine . Une autre méthode implique l'utilisation d'intermédiaires de sulfate cyclique pour obtenir la stéréochimie souhaitée .
Méthodes de Production Industrielle : La production industrielle de l'érythrosphingosine implique souvent l'extraction de sources naturelles, telles que les tissus animaux, ou par des processus de fermentation utilisant des levures. Le composé extrait est ensuite purifié pour atteindre les niveaux de pureté élevés requis pour diverses applications .
Analyse Des Réactions Chimiques
Types de Réactions : L'érythrosphingosine subit plusieurs types de réactions chimiques, notamment :
Substitution : L'érythrosphingosine peut subir des réactions de substitution pour former divers dérivés, tels que les N-acyl sphingosines (céramides).
Réactifs et Conditions Courants :
Oxydation : Les oxydants courants incluent le peroxyde d'hydrogène et les peracides.
Réduction : Des agents réducteurs tels que le borohydrure de sodium sont souvent utilisés.
Substitution : Les chlorures d'acyle et les anhydrides sont des réactifs couramment utilisés pour les réactions de substitution.
Produits Principaux :
Sphingosine-1-phosphate : Formé par oxydation et joue un rôle dans la signalisation cellulaire.
Dihydrosphingosine : Formé par réduction et a des fonctions biologiques distinctes.
Céramides : Formés par substitution et sont des composants clés des membranes cellulaires.
4. Applications de Recherche Scientifique
L'érythrosphingosine a une large gamme d'applications de recherche scientifique, notamment :
Biologie : Joue un rôle dans les voies de signalisation cellulaire, l'apoptose et la prolifération cellulaire.
5. Mécanisme d'Action
L'érythrosphingosine exerce ses effets par le biais de plusieurs cibles moléculaires et voies :
Inhibition de la Protéine Kinase C : L'érythrosphingosine inhibe la protéine kinase C, qui est impliquée dans divers processus cellulaires, notamment la croissance et la différenciation cellulaires.
Activation de la Protéine Phosphatase 2A : Elle active la protéine phosphatase 2A, qui joue un rôle dans la régulation du cycle cellulaire et de l'apoptose.
Voie du Sphingosine-1-Phosphate : L'érythrosphingosine peut être convertie en sphingosine-1-phosphate, qui se lie aux récepteurs couplés aux protéines G et régule la survie, la prolifération et la migration cellulaires.
Applications De Recherche Scientifique
Erythrosphingosine has a wide range of scientific research applications, including:
Comparaison Avec Des Composés Similaires
L'érythrosphingosine est souvent comparée à d'autres bases sphingoïdes, telles que :
Sphinganine : Structure similaire mais sans la double liaison présente dans l'érythrosphingosine.
Phytosphingosine : Contient des groupes hydroxyle supplémentaires et se trouve principalement dans les plantes.
Sphingosine-1-Phosphate : Un dérivé phosphorylé de l'érythrosphingosine qui joue un rôle crucial dans la signalisation cellulaire et les réponses immunitaires.
L'érythrosphingosine est unique en raison de sa stéréochimie spécifique et de sa capacité à inhiber la protéine kinase C tout en activant la protéine phosphatase 2A, ce qui en fait un composé précieux dans les applications de recherche et industrielles .
Activité Biologique
Triethoxy-p-tolylsilane (TEpTS) is an organosilicon compound that has garnered attention for its potential biological activities and applications in various fields, including medicinal chemistry, materials science, and biochemistry. This article reviews the biological activity of TEpTS, highlighting its mechanisms of action, pharmacokinetics, and potential therapeutic applications based on recent research findings.
Chemical Structure and Properties
This compound has the molecular formula and is characterized by a silicon atom bonded to a p-tolyl group and three ethoxy groups. This structure imparts unique chemical properties, making it versatile in chemical reactions and interactions with biological systems.
Mechanisms of Biological Activity
- Reactivity with Biological Molecules : The silicon-hydrogen bond in TEpTS allows it to undergo various chemical transformations that can interact with biological molecules. This reactivity can lead to the formation of silanol groups, which may influence cellular processes.
- Modulation of Cellular Functions : Research indicates that TEpTS and its derivatives can modulate cellular functions such as proliferation and apoptosis. For instance, studies have shown that triethoxysilanes can affect tubulin dynamics, which is crucial for cell division and stability .
- Antimitotic Properties : TEpTS has been identified as a potential antimitotic agent, demonstrating the ability to inhibit cell division by interfering with microtubule formation. This property makes it a candidate for further investigation in cancer therapy .
Pharmacokinetics
Organosilicon compounds like TEpTS are generally known for their stability and resistance to metabolic degradation. This stability can impact their bioavailability and efficacy in biological systems. The pharmacokinetic profile of TEpTS suggests that it may persist in biological environments, allowing for sustained action against target cells.
Case Study 1: Antitumor Activity
A study investigated the effects of TEpTS on various cancer cell lines. The results indicated that TEpTS exhibited significant cytotoxicity against breast cancer cells, with an IC50 value indicating potent activity. The mechanism was attributed to the disruption of microtubule dynamics, leading to cell cycle arrest at the G2/M phase .
Case Study 2: Synthesis of Biologically Active Compounds
TEpTS has been utilized as a precursor in the synthesis of novel biphenyl derivatives with enhanced biological activity. The Hiyama coupling reaction involving TEpTS demonstrated promising yields for compounds that displayed antitumor properties in preliminary assays .
Comparative Analysis of Related Compounds
Compound Name | Structure | Biological Activity |
---|---|---|
This compound | C₁₃H₁₈O₃Si | Antimitotic properties; influences tubulin dynamics |
Trimethoxy(p-tolyl)silane | C₇H₉O₃Si | Used for surface modifications; less studied biologically |
Tetra-p-tolylsilane | C₂₈H₃₂Si | Exhibits enhanced reactivity; potential in drug design |
Propriétés
IUPAC Name |
triethoxy-(4-methylphenyl)silane | |
---|---|---|
Source | PubChem | |
URL | https://pubchem.ncbi.nlm.nih.gov | |
Description | Data deposited in or computed by PubChem | |
InChI |
InChI=1S/C13H22O3Si/c1-5-14-17(15-6-2,16-7-3)13-10-8-12(4)9-11-13/h8-11H,5-7H2,1-4H3 | |
Source | PubChem | |
URL | https://pubchem.ncbi.nlm.nih.gov | |
Description | Data deposited in or computed by PubChem | |
InChI Key |
PADYPAQRESYCQZ-UHFFFAOYSA-N | |
Source | PubChem | |
URL | https://pubchem.ncbi.nlm.nih.gov | |
Description | Data deposited in or computed by PubChem | |
Canonical SMILES |
CCO[Si](C1=CC=C(C=C1)C)(OCC)OCC | |
Source | PubChem | |
URL | https://pubchem.ncbi.nlm.nih.gov | |
Description | Data deposited in or computed by PubChem | |
Molecular Formula |
C13H22O3Si | |
Source | PubChem | |
URL | https://pubchem.ncbi.nlm.nih.gov | |
Description | Data deposited in or computed by PubChem | |
DSSTOX Substance ID |
DTXSID70405407 | |
Record name | Triethoxy-p-tolylsilane | |
Source | EPA DSSTox | |
URL | https://comptox.epa.gov/dashboard/DTXSID70405407 | |
Description | DSSTox provides a high quality public chemistry resource for supporting improved predictive toxicology. | |
Molecular Weight |
254.40 g/mol | |
Source | PubChem | |
URL | https://pubchem.ncbi.nlm.nih.gov | |
Description | Data deposited in or computed by PubChem | |
CAS No. |
18412-57-2 | |
Record name | Triethoxy-p-tolylsilane | |
Source | EPA DSSTox | |
URL | https://comptox.epa.gov/dashboard/DTXSID70405407 | |
Description | DSSTox provides a high quality public chemistry resource for supporting improved predictive toxicology. | |
Record name | Triethoxy(p-tolyl)silane | |
Source | European Chemicals Agency (ECHA) | |
URL | https://echa.europa.eu/information-on-chemicals | |
Description | The European Chemicals Agency (ECHA) is an agency of the European Union which is the driving force among regulatory authorities in implementing the EU's groundbreaking chemicals legislation for the benefit of human health and the environment as well as for innovation and competitiveness. | |
Explanation | Use of the information, documents and data from the ECHA website is subject to the terms and conditions of this Legal Notice, and subject to other binding limitations provided for under applicable law, the information, documents and data made available on the ECHA website may be reproduced, distributed and/or used, totally or in part, for non-commercial purposes provided that ECHA is acknowledged as the source: "Source: European Chemicals Agency, http://echa.europa.eu/". Such acknowledgement must be included in each copy of the material. ECHA permits and encourages organisations and individuals to create links to the ECHA website under the following cumulative conditions: Links can only be made to webpages that provide a link to the Legal Notice page. | |
Retrosynthesis Analysis
AI-Powered Synthesis Planning: Our tool employs the Template_relevance Pistachio, Template_relevance Bkms_metabolic, Template_relevance Pistachio_ringbreaker, Template_relevance Reaxys, Template_relevance Reaxys_biocatalysis model, leveraging a vast database of chemical reactions to predict feasible synthetic routes.
One-Step Synthesis Focus: Specifically designed for one-step synthesis, it provides concise and direct routes for your target compounds, streamlining the synthesis process.
Accurate Predictions: Utilizing the extensive PISTACHIO, BKMS_METABOLIC, PISTACHIO_RINGBREAKER, REAXYS, REAXYS_BIOCATALYSIS database, our tool offers high-accuracy predictions, reflecting the latest in chemical research and data.
Strategy Settings
Precursor scoring | Relevance Heuristic |
---|---|
Min. plausibility | 0.01 |
Model | Template_relevance |
Template Set | Pistachio/Bkms_metabolic/Pistachio_ringbreaker/Reaxys/Reaxys_biocatalysis |
Top-N result to add to graph | 6 |
Feasible Synthetic Routes
Q1: How does Triethoxy(p-tolyl)silane influence the properties of the resulting silica xerogels?
A1: Triethoxy(p-tolyl)silane (MPhTEOS) plays a crucial role in determining the final properties of the hybrid silica xerogels. When co-condensed with tetraethoxysilane (TEOS), the MPhTEOS introduces organic functionality into the silica network. This organic incorporation directly impacts the material's porosity, hydrophilicity, and local order []. By varying the molar percentage of MPhTEOS during synthesis, researchers can fine-tune these properties. For example, higher concentrations of MPhTEOS might lead to increased hydrophobicity due to the presence of the p-tolyl group.
Avertissement et informations sur les produits de recherche in vitro
Veuillez noter que tous les articles et informations sur les produits présentés sur BenchChem sont destinés uniquement à des fins informatives. Les produits disponibles à l'achat sur BenchChem sont spécifiquement conçus pour des études in vitro, qui sont réalisées en dehors des organismes vivants. Les études in vitro, dérivées du terme latin "in verre", impliquent des expériences réalisées dans des environnements de laboratoire contrôlés à l'aide de cellules ou de tissus. Il est important de noter que ces produits ne sont pas classés comme médicaments et n'ont pas reçu l'approbation de la FDA pour la prévention, le traitement ou la guérison de toute condition médicale, affection ou maladie. Nous devons souligner que toute forme d'introduction corporelle de ces produits chez les humains ou les animaux est strictement interdite par la loi. Il est essentiel de respecter ces directives pour assurer la conformité aux normes légales et éthiques en matière de recherche et d'expérimentation.