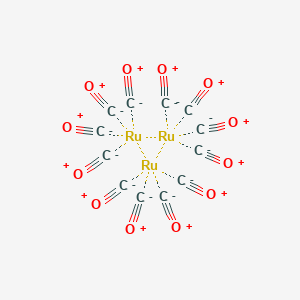
Triruténium, dodécacarbonyle-
- Cliquez sur DEMANDE RAPIDE pour recevoir un devis de notre équipe d'experts.
- Avec des produits de qualité à un prix COMPÉTITIF, vous pouvez vous concentrer davantage sur votre recherche.
Vue d'ensemble
Description
Ruthenium, dodecacarbonyltri-, triangulo, also known as triruthenium dodecacarbonyl, is a coordination compound with the formula Ru₃(CO)₁₂. It consists of a triangular arrangement of three ruthenium atoms, each bonded to four carbon monoxide molecules. This compound appears as dark orange crystals and is soluble in nonpolar solvents but not in water .
Applications De Recherche Scientifique
Triruthenium dodecacarbonyl has numerous applications in scientific research:
Mécanisme D'action
Target of Action
The primary target of Ruthenium, dodecacarbonyltri-, triangulo, also known as Triruthenium dodecacarbonyl, is a coordination compound that consists of a triangle of ruthenium atoms . Each of these ruthenium atoms is bonded to four carbon monoxide molecules . This compound appears as dark orange crystals .
Mode of Action
The compound interacts with its targets through a coordination bond, where each ruthenium atom in the triangle bonds with four carbon monoxide molecules . This results in a complex with D3h symmetry , consisting of an equilateral triangle of Ru atoms, each of which bears two axial and two equatorial CO ligands .
Biochemical Pathways
It’s known that the compound is used in the synthesis of other ruthenium complexes , many with CO combined with other ligands such as alkenes . This suggests that it may play a role in various biochemical reactions involving these complexes.
Result of Action
The primary use for Ruthenium, dodecacarbonyltri-, triangulo is the synthesis of other ruthenium complexes . It has also been used for making ruthenium films deposited on silicon substrates that form semiconductors . Therefore, the molecular and cellular effects of this compound’s action are likely related to these applications.
Action Environment
The action, efficacy, and stability of Ruthenium, dodecacarbonyltri-, triangulo can be influenced by various environmental factors. For instance, the compound is synthesized via the base-catalyzed liquid-phase reaction of ruthenium trichloride with CO . Therefore, the presence of a base and CO, as well as the phase of the reaction, can influence the compound’s action. Additionally, the compound has been made under high CO pressure , suggesting that pressure can also affect its action and stability.
Méthodes De Préparation
Triruthenium dodecacarbonyl is synthesized through several methods:
Base-Catalyzed Liquid-Phase Reaction: Ruthenium trichloride (RuCl₃) reacts with carbon monoxide (CO) in the presence of a base.
High CO Pressure Method: Ruthenium stearate dissolved in cyclohexane or a mixture of RuCl₃ and metallic zinc in ethanol under high CO pressure.
Methanol Reaction: A reaction involving ruthenium trichloride, carbon monoxide, and methanol can also produce this compound.
Analyse Des Réactions Chimiques
Triruthenium dodecacarbonyl undergoes various chemical reactions:
Oxidation and Reduction: It can be oxidized or reduced depending on the reagents and conditions used.
Substitution Reactions: Common reagents include phosphines and alkenes, which can replace one or more carbon monoxide ligands.
High-Pressure CO Reaction: High pressures of CO convert the cluster to monomeric ruthenium pentacarbonyl, which can revert to the parent cluster upon standing.
Comparaison Avec Des Composés Similaires
Triruthenium dodecacarbonyl is often compared to similar metal carbonyl clusters:
Triosmium dodecacarbonyl (Os₃(CO)₁₂): Similar structure but with osmium atoms.
Triiron dodecacarbonyl (Fe₃(CO)₁₂): Contains iron atoms and has bridging CO ligands, resulting in different symmetry (C₂v).
These comparisons highlight the unique properties of triruthenium dodecacarbonyl, particularly its stability and reactivity in various chemical environments.
Propriétés
Numéro CAS |
15243-33-1 |
---|---|
Formule moléculaire |
C12O12Ru3 |
Poids moléculaire |
639.3 g/mol |
Nom IUPAC |
carbon monoxide;ruthenium |
InChI |
InChI=1S/12CO.3Ru/c12*1-2;;; |
Clé InChI |
NQZFAUXPNWSLBI-UHFFFAOYSA-N |
SMILES |
[C-]#[O+].[C-]#[O+].[C-]#[O+].[C-]#[O+].[C-]#[O+].[C-]#[O+].[C-]#[O+].[C-]#[O+].[C-]#[O+].[C-]#[O+].[C-]#[O+].[C-]#[O+].[Ru].[Ru].[Ru] |
SMILES canonique |
[C-]#[O+].[C-]#[O+].[C-]#[O+].[C-]#[O+].[C-]#[O+].[C-]#[O+].[C-]#[O+].[C-]#[O+].[C-]#[O+].[C-]#[O+].[C-]#[O+].[C-]#[O+].[Ru].[Ru].[Ru] |
Key on ui other cas no. |
15243-33-1 |
Description physique |
Orange crystals; [MSDSonline] |
Pictogrammes |
Irritant |
Origine du produit |
United States |
Q1: What are the key characteristics of Ruthenium, dodecacarbonyltri-, triangulo that make it a suitable catalyst?
A1: Ruthenium, dodecacarbonyltri-, triangulo ([Ru3(CO)12]) [] is a versatile catalyst due to its ability to activate various chemical bonds, including carbon-hydrogen (C-H) bonds. [] This activation allows for the functionalization of molecules, making it valuable in organic synthesis. Its solubility in common organic solvents further enhances its applicability in various reaction media. []
Q2: What specific types of reactions does Ruthenium, dodecacarbonyltri-, triangulo catalyze?
A2: Research highlights its efficacy in catalyzing a range of reactions, including:
- Reduction of Amides: It facilitates the conversion of amides to amines using hydrosilanes as reducing agents. [] This reaction pathway offers an alternative to traditional methods and can be significant for synthesizing amine-containing compounds.
- Water Gas Shift Reaction: This reaction, involving the conversion of carbon monoxide and water to carbon dioxide and hydrogen, is crucial for producing hydrogen gas. [] While the provided research focuses on homogeneous catalysis using various metal complexes, Ruthenium-based catalysts are known to be effective in this process.
- Carbon Monoxide Insertion Reactions: Studies demonstrate its role in promoting carbon monoxide insertion into aryl substituents of N-heterocyclic carbene ligands, showcasing its potential in organometallic synthesis. []
- Homogeneous Hydrogenation of Carbon Monoxide: Research indicates its potential as a catalyst in the presence of iodide promoters for this reaction, highlighting its versatility in different catalytic systems. []
Q3: Can you provide examples of its application in organic synthesis?
A3: One prominent example is the catalytic reduction of amides to amines using Ruthenium, dodecacarbonyltri-, triangulo and hydrosilanes. [] This method provides a milder and potentially more selective route compared to conventional methods.
Avertissement et informations sur les produits de recherche in vitro
Veuillez noter que tous les articles et informations sur les produits présentés sur BenchChem sont destinés uniquement à des fins informatives. Les produits disponibles à l'achat sur BenchChem sont spécifiquement conçus pour des études in vitro, qui sont réalisées en dehors des organismes vivants. Les études in vitro, dérivées du terme latin "in verre", impliquent des expériences réalisées dans des environnements de laboratoire contrôlés à l'aide de cellules ou de tissus. Il est important de noter que ces produits ne sont pas classés comme médicaments et n'ont pas reçu l'approbation de la FDA pour la prévention, le traitement ou la guérison de toute condition médicale, affection ou maladie. Nous devons souligner que toute forme d'introduction corporelle de ces produits chez les humains ou les animaux est strictement interdite par la loi. Il est essentiel de respecter ces directives pour assurer la conformité aux normes légales et éthiques en matière de recherche et d'expérimentation.