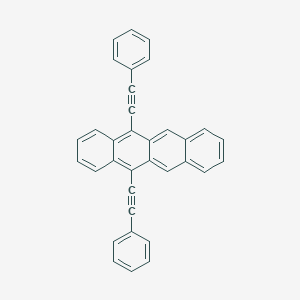
5,12-Bis(phenylethynyl)naphthacene
Vue d'ensemble
Description
5,12-Bis(phenylethynyl)naphthacene is a fluorescent dye known for its ability to emit orange light. It belongs to the class of polycyclic aromatic hydrocarbons and is used in various applications, including lightsticks . The compound has a chemical formula of C₃₄H₂₀ and a molar mass of 428.52 g/mol .
Méthodes De Préparation
Synthetic Routes and Reaction Conditions: 5,12-Bis(phenylethynyl)naphthacene can be synthesized through carbon-carbon coupling reactions. One common method involves the use of lithium amide in anhydrous dioxane, followed by
Activité Biologique
5,12-Bis(phenylethynyl)naphthacene (BPEN) is a polycyclic aromatic hydrocarbon (PAH) that has garnered attention for its unique photophysical properties and potential applications in various fields, including organic electronics and biomedicine. This article explores the biological activity of BPEN, focusing on its mechanisms of action, biochemical interactions, and relevant case studies.
Chemical Structure and Properties
BPEN consists of a naphthacene core with two phenylethynyl substituents at the 5 and 12 positions. This structure contributes to its optical properties, including strong fluorescence and the ability to undergo energy transfer processes.
Target Interactions
BPEN primarily interacts with biological molecules through non-covalent interactions such as π-π stacking and C–H···π interactions. These interactions can influence cellular processes by modulating the activity of enzymes and receptors. BPEN's ability to absorb light and emit fluorescence makes it a candidate for applications in bioimaging and phototherapy.
Biochemical Pathways
BPEN has been shown to affect several biochemical pathways:
- Cell Signaling : BPEN can influence cell signaling pathways, potentially altering gene expression and cellular metabolism.
- Enzyme Modulation : It may act as an inhibitor or activator of specific enzymes, impacting metabolic processes within cells.
- Oxidative Stress Response : BPEN interacts with cellular components involved in oxidative stress responses, suggesting a role in protecting against oxidative damage.
Pharmacokinetics
BPEN exhibits low solubility in water but can dissolve in organic solvents. Its pharmacokinetic profile indicates that it can be effectively utilized in various formulations for biomedical applications.
Dosage Effects
Research indicates that BPEN's effects vary significantly with dosage. At low concentrations, it may serve as a fluorescence probe without inducing significant cytotoxicity. Conversely, higher concentrations could lead to adverse effects on cell viability.
Case Studies
- Fluorescence Imaging : In a study utilizing BPEN as a fluorescent marker, researchers demonstrated its effectiveness in imaging cellular structures due to its high quantum yield and stability under physiological conditions. This property makes it suitable for tracking biological processes in real-time.
- Photodynamic Therapy : BPEN has been explored for use in photodynamic therapy (PDT). When activated by light, it generates reactive oxygen species (ROS), which can induce apoptosis in cancer cells. This application highlights BPEN's potential as an anticancer agent.
Comparative Analysis with Related Compounds
The following table compares BPEN with other similar compounds based on their biological activity and applications:
Compound | Structure Type | Key Application | Fluorescence Yield | Cytotoxicity Level |
---|---|---|---|---|
This compound | Polycyclic Aromatic Hydrocarbon | Bioimaging, PDT | High | Low at low doses |
Rubrene | Tetracene Derivative | Organic Electronics | Very High | Moderate |
9,10-Bis(phenylethynyl)anthracene | Anthracene Derivative | Photonic Devices | High | Low |
Propriétés
IUPAC Name |
5,12-bis(2-phenylethynyl)tetracene | |
---|---|---|
Source | PubChem | |
URL | https://pubchem.ncbi.nlm.nih.gov | |
Description | Data deposited in or computed by PubChem | |
InChI |
InChI=1S/C34H20/c1-3-11-25(12-4-1)19-21-31-29-17-9-10-18-30(29)32(22-20-26-13-5-2-6-14-26)34-24-28-16-8-7-15-27(28)23-33(31)34/h1-18,23-24H | |
Source | PubChem | |
URL | https://pubchem.ncbi.nlm.nih.gov | |
Description | Data deposited in or computed by PubChem | |
InChI Key |
OUHYGBCAEPBUNA-UHFFFAOYSA-N | |
Source | PubChem | |
URL | https://pubchem.ncbi.nlm.nih.gov | |
Description | Data deposited in or computed by PubChem | |
Canonical SMILES |
C1=CC=C(C=C1)C#CC2=C3C=CC=CC3=C(C4=CC5=CC=CC=C5C=C42)C#CC6=CC=CC=C6 | |
Source | PubChem | |
URL | https://pubchem.ncbi.nlm.nih.gov | |
Description | Data deposited in or computed by PubChem | |
Molecular Formula |
C34H20 | |
Source | PubChem | |
URL | https://pubchem.ncbi.nlm.nih.gov | |
Description | Data deposited in or computed by PubChem | |
DSSTOX Substance ID |
DTXSID3066425 | |
Record name | Naphthacene, 5,12-bis(phenylethynyl)- | |
Source | EPA DSSTox | |
URL | https://comptox.epa.gov/dashboard/DTXSID3066425 | |
Description | DSSTox provides a high quality public chemistry resource for supporting improved predictive toxicology. | |
Molecular Weight |
428.5 g/mol | |
Source | PubChem | |
URL | https://pubchem.ncbi.nlm.nih.gov | |
Description | Data deposited in or computed by PubChem | |
CAS No. |
18826-29-4 | |
Record name | 5,12-Bis(2-phenylethynyl)naphthacene | |
Source | CAS Common Chemistry | |
URL | https://commonchemistry.cas.org/detail?cas_rn=18826-29-4 | |
Description | CAS Common Chemistry is an open community resource for accessing chemical information. Nearly 500,000 chemical substances from CAS REGISTRY cover areas of community interest, including common and frequently regulated chemicals, and those relevant to high school and undergraduate chemistry classes. This chemical information, curated by our expert scientists, is provided in alignment with our mission as a division of the American Chemical Society. | |
Explanation | The data from CAS Common Chemistry is provided under a CC-BY-NC 4.0 license, unless otherwise stated. | |
Record name | 5,12-Bis(phenethynyl)naphthacene | |
Source | ChemIDplus | |
URL | https://pubchem.ncbi.nlm.nih.gov/substance/?source=chemidplus&sourceid=0018826294 | |
Description | ChemIDplus is a free, web search system that provides access to the structure and nomenclature authority files used for the identification of chemical substances cited in National Library of Medicine (NLM) databases, including the TOXNET system. | |
Record name | Naphthacene, 5,12-bis(2-phenylethynyl)- | |
Source | EPA Chemicals under the TSCA | |
URL | https://www.epa.gov/chemicals-under-tsca | |
Description | EPA Chemicals under the Toxic Substances Control Act (TSCA) collection contains information on chemicals and their regulations under TSCA, including non-confidential content from the TSCA Chemical Substance Inventory and Chemical Data Reporting. | |
Record name | Naphthacene, 5,12-bis(phenylethynyl)- | |
Source | EPA DSSTox | |
URL | https://comptox.epa.gov/dashboard/DTXSID3066425 | |
Description | DSSTox provides a high quality public chemistry resource for supporting improved predictive toxicology. | |
Record name | 5,12-bis(phenylethynyl)naphthacene | |
Source | European Chemicals Agency (ECHA) | |
URL | https://echa.europa.eu/substance-information/-/substanceinfo/100.038.717 | |
Description | The European Chemicals Agency (ECHA) is an agency of the European Union which is the driving force among regulatory authorities in implementing the EU's groundbreaking chemicals legislation for the benefit of human health and the environment as well as for innovation and competitiveness. | |
Explanation | Use of the information, documents and data from the ECHA website is subject to the terms and conditions of this Legal Notice, and subject to other binding limitations provided for under applicable law, the information, documents and data made available on the ECHA website may be reproduced, distributed and/or used, totally or in part, for non-commercial purposes provided that ECHA is acknowledged as the source: "Source: European Chemicals Agency, http://echa.europa.eu/". Such acknowledgement must be included in each copy of the material. ECHA permits and encourages organisations and individuals to create links to the ECHA website under the following cumulative conditions: Links can only be made to webpages that provide a link to the Legal Notice page. | |
Record name | 5,12-Bis(phenethynyl)naphthacene | |
Source | FDA Global Substance Registration System (GSRS) | |
URL | https://gsrs.ncats.nih.gov/ginas/app/beta/substances/MKZ3D4QP9D | |
Description | The FDA Global Substance Registration System (GSRS) enables the efficient and accurate exchange of information on what substances are in regulated products. Instead of relying on names, which vary across regulatory domains, countries, and regions, the GSRS knowledge base makes it possible for substances to be defined by standardized, scientific descriptions. | |
Explanation | Unless otherwise noted, the contents of the FDA website (www.fda.gov), both text and graphics, are not copyrighted. They are in the public domain and may be republished, reprinted and otherwise used freely by anyone without the need to obtain permission from FDA. Credit to the U.S. Food and Drug Administration as the source is appreciated but not required. | |
Avertissement et informations sur les produits de recherche in vitro
Veuillez noter que tous les articles et informations sur les produits présentés sur BenchChem sont destinés uniquement à des fins informatives. Les produits disponibles à l'achat sur BenchChem sont spécifiquement conçus pour des études in vitro, qui sont réalisées en dehors des organismes vivants. Les études in vitro, dérivées du terme latin "in verre", impliquent des expériences réalisées dans des environnements de laboratoire contrôlés à l'aide de cellules ou de tissus. Il est important de noter que ces produits ne sont pas classés comme médicaments et n'ont pas reçu l'approbation de la FDA pour la prévention, le traitement ou la guérison de toute condition médicale, affection ou maladie. Nous devons souligner que toute forme d'introduction corporelle de ces produits chez les humains ou les animaux est strictement interdite par la loi. Il est essentiel de respecter ces directives pour assurer la conformité aux normes légales et éthiques en matière de recherche et d'expérimentation.