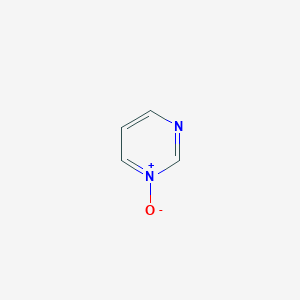
Pyrimidine N-oxide
Vue d'ensemble
Description
Pyrimidine N-oxide is a heterocyclic aromatic compound derived from pyrimidine (a six-membered ring with two nitrogen atoms at positions 1 and 3) through oxidation of one of its nitrogen atoms. This modification introduces a polarized N–O bond, enhancing its reactivity and utility in synthetic chemistry. Pyrimidine N-oxides are synthesized via oxidation of pyrimidine precursors using agents like hydrogen peroxide or m-chloroperbenzoic acid (MCPBA), though hydrolysis and decomposition often limit yields . A novel method employs carboxamide oximes and 1,3-dicarbonyl compounds under trifluoroacetic acid catalysis, offering moderate to high yields and improved stability . These compounds serve as intermediates in pharmaceuticals (e.g., acromelic acid, re~enine) and agrochemicals, and function as ligands, catalysts, or protecting groups .
Méthodes De Préparation
Synthetic Routes and Reaction Conditions: Pyrimidine N-oxide can be synthesized through the oxidation of pyrimidine using various oxidizing agents. Common methods include:
Oxidation with Peracids: Peracetic acid and perbenzoic acid are frequently used oxidizing agents for the preparation of this compound.
Urea-Hydrogen Peroxide Complex: This method involves the use of a urea-hydrogen peroxide complex, which is a stable and easily handled reagent.
Sodium Percarbonate: This reagent, in the presence of catalysts such as methylrhenium trioxide, can efficiently oxidize pyrimidine to its N-oxide form.
Industrial Production Methods: Industrial production of this compound typically involves continuous flow processes using packed-bed microreactors. These methods offer higher efficiency, safety, and environmental benefits compared to traditional batch reactors .
Analyse Des Réactions Chimiques
Photochemical Reactions
Pyrimidine N-oxides undergo photolysis to form intermediates such as oxaziridines and diazo ketones. These reactions are highly dependent on substituent effects and reaction conditions:
Key Findings:
-
Deoxygenation : UV irradiation in benzene yields deoxygenated pyrimidines while generating phenol via atomic oxygen transfer to solvent .
-
Oxaziridine Formation : Substituents at the 4-position (e.g., methyl groups) stabilize transient oxaziridine intermediates, which isomerize to 1,2-oxazepines (Fig. 1A) .
-
Solvent Effects : Methanol or ethanol as solvents lead to aldehyde byproducts via oxygen-mediated oxidation .
Table 1: Photolysis Products of 4-Substituted Pyrimidine N-Oxides
Substituent (R) | Major Product | Minor Product | Yield (%) |
---|---|---|---|
CH₃ | 1,2-Oxazepine | 2-Formylpyrrole | 78 |
Cl | Deoxygenated pyrimidine | Phenol | 65 |
NO₂ | Nitroso compound | - | 42 |
Thermal Rearrangements
The Boekelheide rearrangement of pyrimidine N-oxides involves -sigmatropic shifts or radical intermediates, producing 4-acetoxymethyl derivatives.
Mechanism and Evidence:
-
Radical Pathway : Trapping experiments with TEMPO confirm the involvement of (pyrimidin-4-yl)methyl radicals .
-
Substituent Effects : Electron-donating groups (e.g., -OAc) enhance rearrangement efficiency, while electron-withdrawing groups (-NO₂) favor side reactions .
Table 2: Boekelheide Rearrangement Outcomes
Starting Material | Conditions | Major Product | Side Products |
---|---|---|---|
4-Methylpyrimidine N-oxide | Ac₂O, 100°C, 12h | 4-Acetoxymethylpyrimidine | Dimers (15%) |
4-Nitrothis compound | Ac₂O, 120°C, 6h | 4-Nitrobenzaldehyde | Pyrimidine decomposition |
Nucleophilic Substitution Reactions
Pyrimidine N-oxides participate in SNAr reactions at accelerated rates compared to non-oxidized pyrimidines, particularly at the 2-, 4-, and 6-positions .
Notable Examples:
-
Chlorination : Treatment with POCl₃ yields 4-chloropyrimidines (85–92% yield) .
-
Amination : Reactivity with ammonia at 150°C produces 4-aminopyrimidines, though competing deoxygenation occurs in polar aprotic solvents .
Equation 1 :
Reactions with Arenesulfonyl Azides
Pyrimidine N-oxides react thermally with arenesulfonyl azides to form tetrazolo[1,5-a]pyrimidines, a class of bioactive heterocycles .
Experimental Insights:
-
Scope : Condensed pyrimidine N-oxides (e.g., quinazoline N-oxide) yield fused tetrazoloazines (Fig. 1B) .
-
Limitations : Electron-deficient pyrimidine rings (e.g., 5-nitro substituents) exhibit reduced reactivity due to decreased nucleophilicity .
Figure 1 :
(A) Photochemical pathway for oxaziridine formation .
(B) Tetrazoloazine synthesis via azido transfer .
N-Oxide Reduction and Functionalization
Pyrimidine N-oxides are precursors to functionalized pyrimidines via deoxygenation or cross-coupling:
Applications De Recherche Scientifique
Pharmaceutical Applications
Pyrimidine N-oxide derivatives have shown promise in the pharmaceutical industry, particularly in the development of anticancer agents and other therapeutic compounds.
1.1 Anticancer Activity
Recent studies have indicated that certain this compound derivatives exhibit cytotoxic effects against cancer cell lines. For instance, a series of novel pyrimidine N-oxides demonstrated significant activity against breast adenocarcinoma cells, highlighting their potential as anticancer agents . The structural modification of these compounds can enhance their efficacy and selectivity.
1.2 Antihypertensive Properties
Minoxidil, a well-known antihypertensive drug, contains a this compound moiety. Its effectiveness in treating hypertension and promoting hair growth has made it a subject of extensive research . The N-oxide functionality contributes to its pharmacological activity, making it a valuable scaffold for drug design.
1.3 Antiviral Activity
Pyrimidine N-oxides have been explored for their antiviral properties. Some derivatives have shown inhibitory effects against viruses such as SARS-CoV and feline coronavirus, suggesting their potential as antiviral agents . The mechanism of action often involves interference with viral replication processes.
Material Science Applications
Pyrimidine N-oxides are also being investigated for their applications in materials science, particularly in the development of functional materials.
2.1 Fluorescent Materials
The unique electronic properties of pyrimidine N-oxides allow them to be utilized in the creation of fluorescent materials. A study reported the synthesis of π-conjugated systems based on pyrimidine N-oxides that exhibited fluorescence in the visible region, making them suitable for bioimaging applications . The fluorescence properties are attributed to intramolecular charge transfer processes facilitated by the N-oxide group.
2.2 Chemosensors
Pyrimidine N-oxides have been developed as chemosensors for detecting metal ions and acids. The ability to undergo changes in fluorescence upon interaction with specific analytes makes them valuable for environmental monitoring and analytical chemistry .
Bioimaging Applications
The biocompatibility and fluorescent properties of pyrimidine N-oxides position them as promising candidates for bioimaging probes.
3.1 Intracellular Imaging
Research has demonstrated that certain this compound derivatives can penetrate cellular membranes and provide fluorescence signals within cells. This capability is crucial for real-time imaging of biological processes, aiding in drug delivery studies and cellular behavior analysis .
3.2 Sensing Applications
The zwitterionic nature of pyrimidine N-oxides enhances their solubility and interaction with biological molecules, making them effective sensors for detecting biomolecules or changes in cellular environments . Their application in sensing is particularly relevant in the context of disease diagnosis and monitoring.
Case Studies
Mécanisme D'action
The mechanism of action of pyrimidine N-oxide involves its ability to participate in various chemical reactions due to the presence of the N-oxide group. This group can act as an electron-withdrawing substituent, influencing the reactivity of the pyrimidine ring. In biological systems, this compound can interact with molecular targets such as enzymes and receptors, potentially leading to therapeutic effects .
Comparaison Avec Des Composés Similaires
Pyridine N-Oxide
Structural Differences : Pyridine N-oxide has a single nitrogen atom in its six-membered ring, while pyrimidine N-oxide has two nitrogen atoms.
Reactivity :
- Methylation : this compound favors O-methylation kinetically (ΔG‡ = 97–105 kJ/mol) despite N-methylation being thermodynamically preferred (ΔG‡ = 108–118 kJ/mol) . In contrast, pyridine N-oxide undergoes N-methylation more readily due to less steric hindrance and higher nucleophilicity at nitrogen.
- Cross-Coupling : Pyridine N-oxides are more reactive in palladium-catalyzed cross-couplings than pyrimidine N-oxides, as diazine N-oxides (e.g., pyrimidine) suffer from catalyst poisoning by free nitrogen atoms and lower nucleophilicity .
Applications : Pyridine N-oxides are widely used in catalysis and drug design (e.g., voriconazole metabolism involves this compound formation) .
Quinazoline 3-Oxides
Structural Differences : Quinazoline 3-oxides are benzo-fused pyrimidine N-oxides, combining aromatic stability with enhanced electronic properties.
Synthesis : They are synthesized via oxidation of quinazolines but face challenges like ring-opening and low yields, similar to pyrimidine N-oxides .
Reactivity : Quinazoline 3-oxides are more thermodynamically stable than pyrimidine N-oxides due to aromatic fusion, enabling their use in synthesizing benzodiazepines (e.g., chlordiazepoxide) .
Applications : They exhibit bronchodilatory, cardiotonic, and fungicidal activities but are less studied compared to pyrimidine N-oxides .
Diazine N-Oxides (Pyrazine N-Oxide)
Structural Differences : Diazines (e.g., pyrazine) have two nitrogen atoms in a six-membered ring but at positions 1 and 4, unlike pyrimidine (1 and 3).
Reactivity :
- Methylation : this compound shows lower thermodynamic favorability (ΔrG° = +14 to +19 kJ/mol) compared to pyrazine N-oxide (ΔrG° = −5 to −10 kJ/mol). Pyrazine N-oxide also undergoes N-methylation faster (ΔG‡ = 85–90 kJ/mol vs. 97–105 kJ/mol for pyrimidine) .
- Cross-Coupling : Pyrazine N-oxides are more reactive in direct arylation due to higher C–H acidity, whereas pyrimidine N-oxides require copper(I) additives to mitigate catalyst poisoning .
Comparison Table: Key Parameters
Mechanistic Insights and Challenges
- Oxidative Stability : Pyrimidine N-oxides are prone to hydrolysis and decomposition during synthesis, necessitating protective strategies (e.g., Boc protection of intermediates) .
- Regioselectivity: Competing N- and O-methylation pathways in this compound highlight the role of kinetic vs. thermodynamic control. Computational studies (DLPNO-CCSD(T)/M06-2X) validate experimental observations of 7% N-methylation vs. 93% O-methylation .
- Catalytic Limitations : Diazine N-oxides require copper additives in cross-couplings to prevent catalyst deactivation, unlike pyridine N-oxides .
Activité Biologique
Pyrimidine N-oxide is a derivative of pyrimidine, a heterocyclic compound that plays a crucial role in various biological processes. This article focuses on the biological activity of this compound, highlighting its potential applications in medicinal chemistry, its mechanisms of action, and relevant case studies.
Chemical Structure and Properties
This compound features a nitrogen atom in the pyrimidine ring that is oxidized, leading to unique chemical properties. This modification enhances the reactivity of the compound and allows it to participate in various biological interactions. The general structure can be represented as follows:
Biological Activity Overview
Pyrimidine N-oxides exhibit a range of biological activities, including:
- Anticancer Activity : Certain derivatives have shown cytotoxic effects against cancer cell lines.
- Antimicrobial Properties : These compounds possess activity against various pathogens.
- Fluorescent Properties : Some derivatives can be used as fluorescent probes in bioimaging.
Anticancer Activity
Recent studies have demonstrated that specific this compound derivatives exhibit significant cytotoxicity against cancer cells. For instance, three derivatives were found to be effective against breast adenocarcinoma cell lines, indicating their potential as anticancer agents. The mechanism of action may involve interference with cellular processes such as apoptosis and cell cycle regulation .
Compound | Cell Line Tested | IC50 (µM) | Mechanism of Action |
---|---|---|---|
3a | Breast Adenocarcinoma | 15 | Induction of apoptosis |
3b | Lung Cancer | 20 | Cell cycle arrest |
3c | Colon Cancer | 25 | Inhibition of proliferation |
Antimicrobial Activity
Pyrimidine N-oxides also show promise as antimicrobial agents. Their activity has been evaluated against various bacterial strains, including Staphylococcus aureus and Escherichia coli. The structure-activity relationship (SAR) studies indicate that modifications to the pyrimidine ring can enhance antimicrobial efficacy .
Fluorescent Properties
The fluorescent characteristics of pyrimidine N-oxides make them suitable for bioimaging applications. A study highlighted the synthesis of novel π-conjugated systems based on this compound, which exhibited fluorescence in the visible region with emission maxima up to 575 nm. This property is particularly useful for developing fluorescent probes for cellular imaging .
Case Study 1: Cytotoxicity Against Cancer Cells
In a study conducted by Sedenkova et al., several this compound derivatives were synthesized and tested for their cytotoxic effects on breast adenocarcinoma cells. The results indicated that derivative 3a had excellent biocompatibility and could efficiently enter cells, making it a promising candidate for further development as an anticancer drug .
Case Study 2: Antimicrobial Efficacy
Research published in Medicinal Chemistry explored the antimicrobial properties of pyrimidine N-oxides against Staphylococcus aureus. The study revealed that certain derivatives exhibited significant inhibitory effects, suggesting their potential use in treating bacterial infections .
Q & A
Basic Research Questions
Q. What are the common synthetic routes for preparing pyrimidine N-oxides, and how do reaction conditions influence product purity?
Pyrimidine N-oxides are typically synthesized via oxidation of pyrimidine derivatives using agents like hydrogen peroxide (H₂O₂), potassium permanganate (KMnO₄), or TiCl₃ under controlled pH and temperature. For example, pyrimidine rings can be oxidized selectively using H₂O₂ in acetic acid at 60–80°C to yield N-oxide derivatives. Product purity is influenced by stoichiometry, reaction time, and post-synthesis purification (e.g., recrystallization or column chromatography). Trace impurities, such as unreacted starting materials, are minimized by optimizing oxidant equivalents and monitoring reaction progress via TLC or HPLC .
Q. What analytical techniques are recommended for confirming the formation of pyrimidine N-oxide metabolites in pharmacokinetic studies?
Liquid chromatography-mass spectrometry (LC-MS) and ¹H–¹⁵N HMBC NMR are critical for identifying N-oxide metabolites. For instance, TiCl₃ reduction can confirm N-oxide formation by observing peak disappearance in LC-MS chromatograms. Fragmentation patterns in high-resolution MS and isotopic labeling (e.g., ¹⁵N) further validate metabolite structures. Stability studies in simulated biological fluids (e.g., pH 7.4 buffers) are also essential to assess metabolic pathways .
Q. What factors influence the stability of pyrimidine N-oxides under basic conditions, and how can decomposition be mitigated?
Pyrimidine N-oxides undergo covalent hydration in strongly basic media (e.g., 0.3N NaOH), forming hydrated intermediates detectable via ¹H NMR (e.g., singlet at δ 8.08 for H-2). Decomposition is mitigated by avoiding prolonged exposure to high pH and using stabilizing agents like chelators (e.g., EDTA) to prevent metal-catalyzed degradation. Kinetic monitoring via PMR spectroscopy helps identify optimal storage conditions (e.g., refrigerated, inert atmosphere) .
Advanced Research Questions
Q. How does Marcus theory explain the preference for O-methylation over N-methylation in this compound reactions with methylating agents?
Despite N-methylation being thermodynamically favored (ΔrG° = −5.2 kcal/mol for MeOTf), O-methylation is kinetically dominant due to lower activation barriers (ΔG‡(O) = 18.3 vs. ΔG‡(N) = 22.1 kcal/mol). Marcus theory calculations at the DLPNO-CCSD(T)/def2-TZVPPD level reveal that the intrinsic barrier for O-methylation is lower, driven by favorable orbital overlap and solvent effects (e.g., acetonitrile stabilizes transition states). Experimental validation shows ~93% O-methylation vs. 7% N-methylation, aligning with computed ΔG‡ differences .
Q. How can UV-induced oxidation pathways of pyrimidine in aqueous environments inform experimental design for studying prebiotic chemistry?
UV irradiation of pyrimidine in H₂O ice generates hydroxylated derivatives (e.g., 4-hydroxypyrimidine) via barrierless OH radical attack. Computational DFT studies predict regioselectivity (4-position favored by −23.4 kcal/mol), corroborated by HPLC/GC-MS detection of 4-3H-pyrimidone. Contradictions, such as the nondetection of 2-hydroxypyrimidine, suggest instability during sample workup. Experimental design should include cryogenic quenching and derivatization (e.g., silylation) to preserve labile products .
Q. What methodologies resolve contradictions between theoretical predictions and experimental detection of oxidized pyrimidine derivatives?
Discrepancies arise from kinetic vs. thermodynamic control and post-reaction degradation. For example, 2-hydroxypyrimidine is computationally predicted but not detected due to rapid tautomerization or decomposition during warm-up. Multi-technique validation (HPLC, GC-MS, and solid-phase NMR) and isotopic tracing (¹³C/¹⁵N) are recommended. Crossover experiments with isotopically labeled reactants can track reversible pathways and intermediate stability .
Propriétés
IUPAC Name |
1-oxidopyrimidin-1-ium | |
---|---|---|
Source | PubChem | |
URL | https://pubchem.ncbi.nlm.nih.gov | |
Description | Data deposited in or computed by PubChem | |
InChI |
InChI=1S/C4H4N2O/c7-6-3-1-2-5-4-6/h1-4H | |
Source | PubChem | |
URL | https://pubchem.ncbi.nlm.nih.gov | |
Description | Data deposited in or computed by PubChem | |
InChI Key |
OQZGLXOADHKTDN-UHFFFAOYSA-N | |
Source | PubChem | |
URL | https://pubchem.ncbi.nlm.nih.gov | |
Description | Data deposited in or computed by PubChem | |
Canonical SMILES |
C1=CN=C[N+](=C1)[O-] | |
Source | PubChem | |
URL | https://pubchem.ncbi.nlm.nih.gov | |
Description | Data deposited in or computed by PubChem | |
Molecular Formula |
C4H4N2O | |
Source | PubChem | |
URL | https://pubchem.ncbi.nlm.nih.gov | |
Description | Data deposited in or computed by PubChem | |
DSSTOX Substance ID |
DTXSID00168856 | |
Record name | Pyrimidine-1-oxide | |
Source | EPA DSSTox | |
URL | https://comptox.epa.gov/dashboard/DTXSID00168856 | |
Description | DSSTox provides a high quality public chemistry resource for supporting improved predictive toxicology. | |
Molecular Weight |
96.09 g/mol | |
Source | PubChem | |
URL | https://pubchem.ncbi.nlm.nih.gov | |
Description | Data deposited in or computed by PubChem | |
CAS No. |
17043-94-6 | |
Record name | Pyrimidine-1-oxide | |
Source | EPA DSSTox | |
URL | https://comptox.epa.gov/dashboard/DTXSID00168856 | |
Description | DSSTox provides a high quality public chemistry resource for supporting improved predictive toxicology. | |
Record name | Pyrimidine N-oxide | |
Source | European Chemicals Agency (ECHA) | |
URL | https://echa.europa.eu/information-on-chemicals | |
Description | The European Chemicals Agency (ECHA) is an agency of the European Union which is the driving force among regulatory authorities in implementing the EU's groundbreaking chemicals legislation for the benefit of human health and the environment as well as for innovation and competitiveness. | |
Explanation | Use of the information, documents and data from the ECHA website is subject to the terms and conditions of this Legal Notice, and subject to other binding limitations provided for under applicable law, the information, documents and data made available on the ECHA website may be reproduced, distributed and/or used, totally or in part, for non-commercial purposes provided that ECHA is acknowledged as the source: "Source: European Chemicals Agency, http://echa.europa.eu/". Such acknowledgement must be included in each copy of the material. ECHA permits and encourages organisations and individuals to create links to the ECHA website under the following cumulative conditions: Links can only be made to webpages that provide a link to the Legal Notice page. | |
Avertissement et informations sur les produits de recherche in vitro
Veuillez noter que tous les articles et informations sur les produits présentés sur BenchChem sont destinés uniquement à des fins informatives. Les produits disponibles à l'achat sur BenchChem sont spécifiquement conçus pour des études in vitro, qui sont réalisées en dehors des organismes vivants. Les études in vitro, dérivées du terme latin "in verre", impliquent des expériences réalisées dans des environnements de laboratoire contrôlés à l'aide de cellules ou de tissus. Il est important de noter que ces produits ne sont pas classés comme médicaments et n'ont pas reçu l'approbation de la FDA pour la prévention, le traitement ou la guérison de toute condition médicale, affection ou maladie. Nous devons souligner que toute forme d'introduction corporelle de ces produits chez les humains ou les animaux est strictement interdite par la loi. Il est essentiel de respecter ces directives pour assurer la conformité aux normes légales et éthiques en matière de recherche et d'expérimentation.