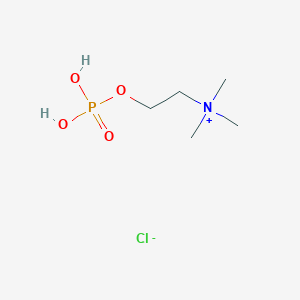
Phosphocholine
Vue d'ensemble
Description
Phosphocholine (PC) is a molecule that plays a crucial role in various biological processes. It is a primary form of organic phosphate transported in plant xylem sap and is involved in the synthesis of phosphatidylcholine, a key component of cell membranes . This compound is also a haptenic molecule, serving as both a precursor and degradation product of choline, and it is found decorating a number of biologics such as lipids and oligosaccharides .
Synthesis Analysis
The synthesis of this compound and its derivatives has been a subject of interest due to its biological significance. Efficient synthetic routes have been developed for macrocyclic bisphosphocholines using a common glycerol synthon, with ring closure achieved through high-dilution Glaser oxidation or olefin metathesis conditions . Additionally, a high-yield synthesis method for phosphatidylcholines from sn-glycero-3-phosphocholine has been described, utilizing 4-pyrrolidinopyridine as a catalyst . For the synthesis of peptides containing phosphocholinated amino acids, a straightforward synthetic strategy using preformed functional amino acid building blocks compatible with standard Fmoc solid-phase peptide synthesis has been established .
Molecular Structure Analysis
This compound consists of a choline molecule bound to a phosphate group. The molecular structure of this compound derivatives can be tailored for specific applications, as seen in the synthesis of enantiomerically pure this compound-acyl-octadecanes, which are structural intermediates between phosphatidylcholine and sphingomyelin . The modular structure of related compounds, such as chiral phosphinooxazolines, allows for variation in the oxazoline ring, backbone, and phosphine moiety to achieve desired steric and electronic properties .
Chemical Reactions Analysis
This compound undergoes various chemical reactions, including phosphitylation, oxidation, and phosphate deprotection, to synthesize high-yield this compound derivatives . The molecule can also be rapidly hydrolyzed outside plant cells, with choline and inorganic phosphate entering the cytosolic compartment to be re-formed into this compound by choline kinase . Furthermore, the synthesis of this compound-containing peptides involves the use of functional amino acid building blocks in a solid-phase synthesis approach .
Physical and Chemical Properties Analysis
This compound's physical and chemical properties are influenced by its amphipathic nature, as seen in the enzyme CTP: this compound cytidylyltransferase, which controls phosphatidylcholine synthesis and exhibits an amphipathic helix that mediates membrane interaction . The transport of this compound in plant cells has been studied using 31P NMR spectroscopy, revealing its accumulation in the cytoplasmic compartment and its role in organic phosphate transport .
Applications De Recherche Scientifique
Lipid Profiling and Metabolism
- Global Lipid Extraction : Phosphocholines are among nine lipid classes constituting 99% of the human serum lipidome. A new one-phase extraction method has been developed for lipid profiling, demonstrating the importance of phosphocholine in lipidomics research (Pellegrino et al., 2014).
Plant Physiology
- This compound Synthesis in Plants : In Spinacia oleracea (spinach), this compound is synthesized through the N-methylation of phosphoethanolamine, indicating its role in plant physiology and response to environmental stress (Smith et al., 2000).
Microbiology
- Bacterial Virulence : The presence of this compound in the lipopolysaccharide of Pasteurella multocida is critical for its virulence, affecting bacterial adhesion and resistance to antimicrobial peptides (Harper et al., 2007).
Biochemical Research
- Post-Translational Modifications : Phosphocholination is identified as an important post-translational modification in pathogenic bacteria, affecting infected host cells (Müller et al., 2014).
- Functionalizing Alcohols with this compound : Development of a stable this compound donor for alcohol functionalization, with applications in drug synthesis like miltefosine production (Xu et al., 2020).
Cancer Research
- Phospholipid Metabolism in Cancer : Research over the past 30 years has focused on phospholipid metabolism in cancer detection, diagnosis, and treatment, with this compound being a key marker (Cohen & Daly, 2022).
Botany
- Role in Plant Development : In Arabidopsis thaliana, this compound is crucial for the biosynthesis of phosphatidylcholine, significantly impacting root system development and cellular integrity (Cruz-Ramírez et al., 2004).
Analytical Chemistry
- Capillary Electrophoresis : Phospholipids, including phosphocholines, enhance the separation of glycans, offering advances in analytical techniques for glycan separation (Luo et al., 2010).
Immunology
- Immunomodulation by this compound : this compound on parasitic antigens can lead to immunomodulation of the host's immune system, offering insights for anti-parasitic drug development and treatment of inflammatory diseases (Grabitzki & Lochnit, 2009).
Mécanisme D'action
Target of Action
Phosphorylcholine primarily targets choline kinase (ChoK), an enzyme that plays a significant role in cell proliferation . The importance of ChoK for the regulation of cell proliferation has been demonstrated, and it is particularly active in ras-transformed cells .
Mode of Action
Phosphorylcholine interacts with its targets, primarily ChoK, resulting in an increased level of phosphorylcholine . This interaction results from a constitutive activation of ChoK . The compound’s hydrophilic polar head group, which is part of some phospholipids, is composed of a negatively charged phosphate bonded to a small, positively charged choline group .
Biochemical Pathways
Phosphorylcholine affects several biochemical pathways. In bacteria containing ChoP-modified glycoconjugates, the Lic-1 pathway mediates the transformation of environmentally-acquired choline to ChoP and its attachment to glycoconjugates . Phosphorylcholine transferase is the only known enzyme involved in ChoP protein modification .
Pharmacokinetics
The pharmacokinetics of Phosphorylcholine involve its absorption, distribution, metabolism, and excretion (ADME). Protein nanocapsules generated by encapsulating proteins with a thin layer of crosslinked phosphorylcholine-based polymer have shown significantly prolonged plasma half-life . This suggests that the compound’s molecular design improves surface biocompatibility and lowers the risk of causing inflammation or thrombosis .
Result of Action
The molecular and cellular effects of Phosphorylcholine’s action are diverse. It plays a vital role in mediating bacterial adhesion and colonization . In the context of interventional cardiology, Phosphorylcholine is used as a synthetic polymer-based coating, applied to drug-eluting stents, to prevent the occurrence of coronary artery restenosis .
Action Environment
The action, efficacy, and stability of Phosphorylcholine can be influenced by various environmental factors. For instance, in the context of interventional cardiology, the compound’s molecular design improves surface biocompatibility and lowers the risk of causing inflammation or thrombosis . This suggests that the compound’s action can be influenced by the physiological environment in which it is applied.
Safety and Hazards
When handling phosphocholine, it is recommended to avoid dust formation, breathing mist, gas or vapors, and contacting with skin and eye. Use personal protective equipment, wear chemical impermeable gloves, ensure adequate ventilation, remove all sources of ignition, evacuate personnel to safe areas, and keep people away from and upwind of spill/leak .
Orientations Futures
Propriétés
IUPAC Name |
trimethyl(2-phosphonooxyethyl)azanium;chloride | |
---|---|---|
Source | PubChem | |
URL | https://pubchem.ncbi.nlm.nih.gov | |
Description | Data deposited in or computed by PubChem | |
InChI |
InChI=1S/C5H14NO4P.ClH/c1-6(2,3)4-5-10-11(7,8)9;/h4-5H2,1-3H3,(H-,7,8,9);1H | |
Source | PubChem | |
URL | https://pubchem.ncbi.nlm.nih.gov | |
Description | Data deposited in or computed by PubChem | |
InChI Key |
PYJNAPOPMIJKJZ-UHFFFAOYSA-N | |
Source | PubChem | |
URL | https://pubchem.ncbi.nlm.nih.gov | |
Description | Data deposited in or computed by PubChem | |
Canonical SMILES |
C[N+](C)(C)CCOP(=O)(O)O.[Cl-] | |
Source | PubChem | |
URL | https://pubchem.ncbi.nlm.nih.gov | |
Description | Data deposited in or computed by PubChem | |
Molecular Formula |
C5H15ClNO4P | |
Source | PubChem | |
URL | https://pubchem.ncbi.nlm.nih.gov | |
Description | Data deposited in or computed by PubChem | |
DSSTOX Substance ID |
DTXSID90910205 | |
Record name | Choline phosphochloride | |
Source | EPA DSSTox | |
URL | https://comptox.epa.gov/dashboard/DTXSID90910205 | |
Description | DSSTox provides a high quality public chemistry resource for supporting improved predictive toxicology. | |
Molecular Weight |
219.60 g/mol | |
Source | PubChem | |
URL | https://pubchem.ncbi.nlm.nih.gov | |
Description | Data deposited in or computed by PubChem | |
CAS RN |
107-73-3 | |
Record name | Phosphocholine | |
Source | CAS Common Chemistry | |
URL | https://commonchemistry.cas.org/detail?cas_rn=107-73-3 | |
Description | CAS Common Chemistry is an open community resource for accessing chemical information. Nearly 500,000 chemical substances from CAS REGISTRY cover areas of community interest, including common and frequently regulated chemicals, and those relevant to high school and undergraduate chemistry classes. This chemical information, curated by our expert scientists, is provided in alignment with our mission as a division of the American Chemical Society. | |
Explanation | The data from CAS Common Chemistry is provided under a CC-BY-NC 4.0 license, unless otherwise stated. | |
Record name | Phosphorylcholine | |
Source | ChemIDplus | |
URL | https://pubchem.ncbi.nlm.nih.gov/substance/?source=chemidplus&sourceid=0000107733 | |
Description | ChemIDplus is a free, web search system that provides access to the structure and nomenclature authority files used for the identification of chemical substances cited in National Library of Medicine (NLM) databases, including the TOXNET system. | |
Record name | Choline phosphochloride | |
Source | EPA DSSTox | |
URL | https://comptox.epa.gov/dashboard/DTXSID90910205 | |
Description | DSSTox provides a high quality public chemistry resource for supporting improved predictive toxicology. | |
Record name | Trimethyl[2-(phosphonooxy)ethyl]ammonium chloride | |
Source | European Chemicals Agency (ECHA) | |
URL | https://echa.europa.eu/substance-information/-/substanceinfo/100.003.197 | |
Description | The European Chemicals Agency (ECHA) is an agency of the European Union which is the driving force among regulatory authorities in implementing the EU's groundbreaking chemicals legislation for the benefit of human health and the environment as well as for innovation and competitiveness. | |
Explanation | Use of the information, documents and data from the ECHA website is subject to the terms and conditions of this Legal Notice, and subject to other binding limitations provided for under applicable law, the information, documents and data made available on the ECHA website may be reproduced, distributed and/or used, totally or in part, for non-commercial purposes provided that ECHA is acknowledged as the source: "Source: European Chemicals Agency, http://echa.europa.eu/". Such acknowledgement must be included in each copy of the material. ECHA permits and encourages organisations and individuals to create links to the ECHA website under the following cumulative conditions: Links can only be made to webpages that provide a link to the Legal Notice page. | |
Record name | PHOSPHORYLCHOLINE CHLORIDE | |
Source | FDA Global Substance Registration System (GSRS) | |
URL | https://gsrs.ncats.nih.gov/ginas/app/beta/substances/96AN057F7A | |
Description | The FDA Global Substance Registration System (GSRS) enables the efficient and accurate exchange of information on what substances are in regulated products. Instead of relying on names, which vary across regulatory domains, countries, and regions, the GSRS knowledge base makes it possible for substances to be defined by standardized, scientific descriptions. | |
Explanation | Unless otherwise noted, the contents of the FDA website (www.fda.gov), both text and graphics, are not copyrighted. They are in the public domain and may be republished, reprinted and otherwise used freely by anyone without the need to obtain permission from FDA. Credit to the U.S. Food and Drug Administration as the source is appreciated but not required. | |
Retrosynthesis Analysis
AI-Powered Synthesis Planning: Our tool employs the Template_relevance Pistachio, Template_relevance Bkms_metabolic, Template_relevance Pistachio_ringbreaker, Template_relevance Reaxys, Template_relevance Reaxys_biocatalysis model, leveraging a vast database of chemical reactions to predict feasible synthetic routes.
One-Step Synthesis Focus: Specifically designed for one-step synthesis, it provides concise and direct routes for your target compounds, streamlining the synthesis process.
Accurate Predictions: Utilizing the extensive PISTACHIO, BKMS_METABOLIC, PISTACHIO_RINGBREAKER, REAXYS, REAXYS_BIOCATALYSIS database, our tool offers high-accuracy predictions, reflecting the latest in chemical research and data.
Strategy Settings
Precursor scoring | Relevance Heuristic |
---|---|
Min. plausibility | 0.01 |
Model | Template_relevance |
Template Set | Pistachio/Bkms_metabolic/Pistachio_ringbreaker/Reaxys/Reaxys_biocatalysis |
Top-N result to add to graph | 6 |
Feasible Synthetic Routes
Q & A
Q1: What is the primary role of phosphocholine in biological systems?
A1: this compound is a vital precursor in the biosynthesis of phosphatidylcholine, a major phospholipid found in cell membranes. [] This pathway involves multiple enzymatic steps, with CTP:this compound cytidylyltransferase (CCT) often acting as a rate-limiting enzyme. [, ]
Q2: How does the structure of this compound relate to its function as a precursor for phosphatidylcholine?
A2: this compound consists of a choline molecule attached to a phosphate group. This structure allows it to be readily activated by CTP, forming CDP-choline, which then reacts with diacylglycerol to yield phosphatidylcholine. [, ]
Q3: Can you elaborate on the role of CCT in the context of this compound metabolism and phosphatidylcholine biosynthesis?
A3: CCT catalyzes the rate-limiting step in the CDP-choline pathway, converting this compound to CDP-choline in the presence of CTP. [, ] Studies on rat CCT alpha, which contains a nuclear localization signal, a central catalytic domain, and lipid-binding and phosphorylation regions, have revealed the importance of specific aspartate residues for its activity. []
Q4: What happens to the activity of CCT when certain aspartate residues within its structure are mutated?
A4: Research indicates that mutating aspartate 86 to alanine in rat CCT alpha significantly reduces the enzyme's ability to produce CDP-choline, while mutations in aspartates 115, 143, and 164 have little effect on catalytic activity. [] This suggests a crucial catalytic role for aspartate 86.
Q5: Besides its role in phosphatidylcholine synthesis, are there other metabolic fates of this compound?
A5: Yes, this compound can be utilized in the synthesis of other important molecules. For instance, in the fungus Penicillium fellutanum, it serves as a precursor for choline-O-sulfate and glycine betaine. []
Q6: How does salt stress affect choline metabolism in plants, particularly concerning this compound?
A6: In spinach, salt stress leads to increased activity of enzymes involved in choline biosynthesis, resulting in a higher accumulation of glycine betaine, an osmoprotectant derived from choline. [] This suggests a mechanism for salt tolerance in plants involving this compound metabolism.
Q7: Can this compound be derived from sources other than de novo synthesis within an organism?
A7: Yes, studies on Penicillium fellutanum show that it can utilize this compound obtained from an extracellular polysaccharide, peptidophosphogalactomannan, as a source for intracellular choline derivatives. [] This highlights the ability of some organisms to scavenge this compound from their environment.
Q8: What is the significance of this compound in bacterial cell walls?
A8: this compound decorates teichoic acids and lipopolysaccharides in bacterial cell walls, playing crucial roles in host cell adhesion, immune evasion, and bacterial persistence. []
Q9: How do bacteria acquire the this compound necessary for cell wall modification?
A9: Bacteria utilize a conserved transporter called LicB for choline uptake, which is then used for this compound synthesis and subsequent cell wall modification. [] This transporter presents a potential target for novel antibacterial therapies.
Q10: Have any inhibitors of LicB been identified?
A10: Yes, studies have shown that synthetic nanobodies (sybodies) and hemicholinium-3 can inhibit the activity of LicB. [] Disrupting LicB function could potentially hinder bacterial virulence by preventing this compound incorporation into cell walls.
Q11: Can you explain the role of this compound in the context of alkylthis compound (APC) drugs?
A11: Alkylphosphocholines, a class of anti-cancer and anti-protozoal drugs, are structural analogs of lysophosphatidylcholine (lyso-PC), a molecule structurally similar to this compound. [] These drugs exert cytotoxic effects by interfering with phospholipid metabolism and other cellular processes.
Q12: Are there specific cellular pathways involved in the cytotoxic effects of alkylphosphocholines?
A12: Research suggests that alkylphosphocholines disrupt various cellular pathways, including:* Phospholipid synthesis: Inhibition of phospholipid synthesis leads to membrane dysfunction. []* Unfolded protein response: Accumulation of misfolded proteins triggers ER stress. []* Oxidative phosphorylation: Disruption of mitochondrial function compromises energy production. []* Lipid rafts: Alterations in membrane microdomains affect signaling and trafficking. []
Q13: How does the structure of alkylphosphocholines relate to their variable effectiveness against different organisms or cell types?
A13: The alkyl chain length and other structural modifications in alkylphosphocholines influence their cellular uptake, subcellular localization, and interactions with specific targets, leading to differences in their efficacy and spectrum of activity. []
Q14: What insights have functional genomic screens provided about the mechanisms of action of alkylphosphocholines?
A14: Screens in Saccharomyces cerevisiae have identified gene deletions conferring resistance to the alkylthis compound drug miltefosine. [] These genes are often involved in vesicular transport, particularly endocytosis and retrograde transport, suggesting a role for these pathways in drug trafficking. []
Q15: What specific cellular components have been implicated in the intracellular transport of alkylphosphocholines?
A15: Functional genomic screens have identified several key players:* Endosomes: Early endosomes and components involved in endosome maturation and sorting are likely involved in alkylthis compound internalization. []* Retrograde transport machinery: Proteins involved in the trafficking of vesicles from endosomes to the Golgi apparatus or vacuole are necessary for delivering alkylphosphocholines to their intracellular targets. []* ER-plasma membrane contact sites: These sites, along with retrograde transport, may facilitate the movement of alkylphosphocholines and related lyso-phospholipid analogs between organelles. []
Q16: Can you provide specific examples of genes and their associated functions that confer resistance to miltefosine?
A16:* STT4 (phosphatidylinositol-4-phosphate synthesis): Mutations in this gene may disrupt proper membrane trafficking and signaling. []* SAC1 (phosphatidylinositol-4-phosphate hydrolysis): Similar to STT4, disruptions in this gene impact membrane dynamics. []* OSH2 (oxysterol binding protein homolog): Involvement in lipid transport and sensing may be crucial for alkylthis compound action. []* ER resident proteins: Alterations in protein folding and quality control within the ER could influence sensitivity to alkylphosphocholines. []* Eisosome components: These membrane domains play a role in endocytosis and lipid homeostasis, potentially influencing alkylthis compound uptake and distribution. []
Q17: What are the implications of these findings for understanding the broader actions of lyso-PtdCho and similar molecules?
A17: The identification of genes involved in alkylthis compound resistance provides a starting point for understanding the intracellular trafficking and targets of lyso-PtdCho and its analogs. [] Further investigations into these pathways may reveal novel therapeutic strategies.
Q18: Can you elaborate on the role of this compound in C-reactive protein (CRP) function?
A18: CRP, an acute-phase protein, exhibits calcium-dependent binding to this compound. [] This interaction is crucial for CRP's ability to recognize and bind to pathogens, activate complement, and participate in the innate immune response. []
Q19: What is the significance of this compound in the context of CRP's interaction with pathogens?
A19: this compound is present in the cell wall and capsular carbohydrates of various bacteria and microorganisms. [] CRP's ability to bind this compound enables it to target these pathogens, marking them for destruction by the immune system.
Q20: Does CRP interact with this compound present in mammalian cell membranes?
A20: Studies have shown that CRP can interact with phosphatidylcholine (PC), a major phospholipid in mammalian cell membranes containing this compound as a headgroup. [] This interaction is thought to contribute to CRP's role in inflammation and tissue damage.
Q21: What evidence supports the interaction between CRP and phosphatidylcholine in membranes?
A21: Several observations point to this interaction:* Flocculation reactions: CRP-positive sera and purified CRP cause flocculation of PC-cholesterol emulsions, indicating an interaction with PC. []* Complement activation: CRP, in the presence of PC-cholesterol emulsions, activates the complement system via the classical pathway. []* Immunofluorescence studies: CRP deposition has been observed in damaged tissues, particularly in association with cell membrane structures. []
Q22: How does cholesterol loading affect phospholipid repair in red blood cells (RBCs)?
A22: In a model of spur cell anemia, which involves increased membrane cholesterol to phospholipid ratios, cholesterol loading inhibits arachidonoyl-CoA:1-palmitoyl-sn-glycero-3-phosphocholine acyl transferase (LAT). []
Q23: What is the function of LAT in red blood cells?
A23: LAT is an enzyme involved in phospholipid repair, specifically in reacylating lysophosphatidylcholine (LPC) to form phosphatidylcholine. []
Q24: How does LAT inhibition relate to the pathology of spur cell anemia?
A24: Inhibition of LAT by cholesterol loading impairs the ability of RBCs to repair their membranes, making them more susceptible to oxidative damage and hemolysis, contributing to the anemia observed in this condition. []
Q25: What evidence links LAT inhibition to impaired phospholipid repair in cholesterol-loaded RBCs?
A25: Studies using radiolabeled arachidonic acid (Ar) have shown that cholesterol-loaded RBCs exhibit:* Decreased Ar incorporation into phospholipids, indicating reduced LAT activity. []* Increased Ar accumulation in acylcarnitine, a fatty acid repair intermediate, further suggesting impaired LAT function. []
Q26: What are the implications of these findings for understanding the role of this compound in membrane dynamics and disease?
A26: This research highlights the importance of maintaining a balanced cholesterol to phospholipid ratio for proper membrane function. [] Dysregulation of this balance, as seen in spur cell anemia, can disrupt phospholipid repair mechanisms, potentially contributing to disease pathogenesis.
Q27: Have there been any studies investigating the use of mass spectrometry for analyzing this compound-containing molecules?
A27: Yes, an improved assay for platelet-activating factor (PAF; 1-O-alkyl-2-acetyl-sn-glycero-3-phosphocholine), a potent signaling molecule derived from this compound, has been developed using HPLC-tandem mass spectrometry (LC-MS/MS). []
Q28: What are the advantages of using LC-MS/MS for PAF analysis?
A28:* High sensitivity: This method can detect as little as 1 pg (1.9 fmol) of PAF, significantly improving upon previous methods. []* Wide linear range: The assay exhibits a linear response up to 1,000 pg of PAF, allowing for quantification over a broad range of concentrations. []* Overcomes interference: This technique mitigates artifacts arising from isobaric lipids that have plagued earlier LC-MS/MS assays for PAF. []
Q29: Has this LC-MS/MS method led to the identification of any novel lipids related to PAF?
A29: Yes, during the development of this assay, researchers detected three new lipid species in human neutrophils, including a potential novel PAF molecular species and two lipids resembling stearoyl-formyl-glycerothis compound and oleoyl-formyl-glycerothis compound. []
Q30: What are the implications of these findings for PAF research and the broader field of lipidomics?
A30: These discoveries underscore the complexity of lipid signaling pathways and highlight the potential for LC-MS/MS to uncover novel lipid mediators. [] Further investigation into these newly identified lipids may reveal their biological functions and potential roles in health and disease.
Q31: How do different phospholipid compositions affect the physical properties of membranes?
A31: The saturation levels of fatty acid chains in phospholipids significantly impact membrane fluidity and phase behavior. [, , , , ] For example, saturated phospholipids like DPPC form more rigid bilayers compared to unsaturated ones like DOPC. [, , ]
Q32: How does the presence of cholesterol influence the properties of phospholipid membranes?
A32: Cholesterol can modulate membrane fluidity by inserting itself between phospholipid molecules, reducing fluidity in liquid-disordered phases and increasing fluidity in gel phases. [, ] It also influences membrane thickness and the formation of lipid rafts. [, ]
Q33: Can you provide specific examples of how different phospholipid compositions affect the interaction of proteins with membranes?
A33:* Human serum albumin (HSA) interacts differently with liposomes composed of saturated versus unsaturated phospholipids. [] HSA penetrates more readily into liposomes made of DPPC and DMPC, which are less hydrated at room temperature due to their higher phase transition temperatures. []* Hen egg white lysozyme (HEWL) interacts with and disrupts liposomes, leading to the release of entrapped molecules like the anticancer drug ellipticine. [] The extent of this interaction depends on the phospholipid composition and the degree of hydration of the liposomes. []* The bacterial mechanosensitive channel MscL, which responds to membrane tension, exhibits thickness-dependent gating behavior. [] MscL opens more readily in thinner membranes composed of DLPC and DMPC compared to thicker membranes of DPPC and DSPC. []
Q34: How do the structural features of phospholipids influence their interactions with proteins?
A34: Factors like the length and saturation of fatty acid chains, the presence of double bonds, and the charge and size of the polar headgroup can affect:* Membrane fluidity and packing: These properties influence the accessibility of protein binding sites and the energy required for protein insertion. [, , ]* Hydrophobic interactions: The length and saturation of fatty acid chains influence the strength of hydrophobic interactions with membrane proteins. [, ]* Electrostatic interactions: The charge on the phospholipid headgroup can mediate electrostatic interactions with charged residues on proteins. []
Q35: What are the implications of these findings for understanding the role of phospholipids in cellular processes and drug delivery?
A35:
* These studies highlight the importance of considering membrane composition when designing liposomal drug delivery systems, as it can influence drug encapsulation, stability, and release. [, ] * Understanding the interplay between phospholipid structure and membrane protein function is crucial for elucidating the mechanisms of various cellular processes, including signaling, transport, and membrane fusion. [, ]
Avertissement et informations sur les produits de recherche in vitro
Veuillez noter que tous les articles et informations sur les produits présentés sur BenchChem sont destinés uniquement à des fins informatives. Les produits disponibles à l'achat sur BenchChem sont spécifiquement conçus pour des études in vitro, qui sont réalisées en dehors des organismes vivants. Les études in vitro, dérivées du terme latin "in verre", impliquent des expériences réalisées dans des environnements de laboratoire contrôlés à l'aide de cellules ou de tissus. Il est important de noter que ces produits ne sont pas classés comme médicaments et n'ont pas reçu l'approbation de la FDA pour la prévention, le traitement ou la guérison de toute condition médicale, affection ou maladie. Nous devons souligner que toute forme d'introduction corporelle de ces produits chez les humains ou les animaux est strictement interdite par la loi. Il est essentiel de respecter ces directives pour assurer la conformité aux normes légales et éthiques en matière de recherche et d'expérimentation.