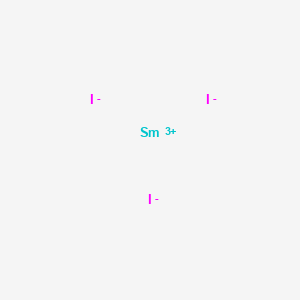
Triiodure de samarium(3+)
- Cliquez sur DEMANDE RAPIDE pour recevoir un devis de notre équipe d'experts.
- Avec des produits de qualité à un prix COMPÉTITIF, vous pouvez vous concentrer davantage sur votre recherche.
Vue d'ensemble
Description
It is a yellow powder that is unstable in air and decomposes in water through hydrolysis . Samarium(III) iodide is commercially available and has been widely used in various organic reactions, such as elimination, conjugate addition, carbonyl reduction, intramolecular cyclization, and nucleophilic substitution reactions .
Applications De Recherche Scientifique
Samarium(III) iodide has a wide range of applications in scientific research, including:
Organic Synthesis: It is used as a reagent in organic synthesis for various transformations, including reductions, substitutions, and eliminations.
Catalysis: Samarium(III) iodide acts as a catalyst in several organic reactions, improving yields and reaction rates.
Material Science: It is used in the preparation of luminescent materials and complexes for optoelectronic devices.
Biomedical Applications: Samarium(III) iodide complexes have been studied for their potential use in biomedical imaging and as therapeutic agents.
Mécanisme D'action
Target of Action
Samarium(3+);triiodide, also known as Samarium (III) iodide, is an inorganic compound that primarily targets metallic samarium . It is used in organic reactions as a catalyst .
Mode of Action
Samarium(3+);triiodide interacts with its target, metallic samarium, through a reduction process . When heated with metallic samarium, it forms samarium diiodide . This interaction results in changes in the compound’s structure and properties.
Biochemical Pathways
The primary biochemical pathway affected by Samarium(3+);triiodide involves the reduction of different functional groups . This includes sulfones and sulfoxides, alkyl and aryl halides, epoxides, phosphine oxides, carbonyls, and conjugated double bonds . The downstream effects of these reactions contribute to the formation of various organic compounds.
Pharmacokinetics
It’s known that the compound decomposes in water, which may affect its bioavailability .
Result of Action
The result of Samarium(3+);triiodide’s action is the formation of samarium diiodide when heated with metallic samarium . This reaction is a key step in various organic reactions where Samarium(3+);triiodide is used as a catalyst .
Action Environment
The action of Samarium(3+);triiodide is influenced by environmental factors such as temperature and presence of water . It is unstable in air and decomposes in water . Therefore, its action, efficacy, and stability are best observed in dry, controlled environments.
Safety and Hazards
Orientations Futures
Samarium(3+);triiodide has found copious applications in organic synthesis . Its inherent strong reducing ability, together with external additives, enable tunable reactivity and endow it with powerful reactivity and impressive chemoselectivity . As a result, it has been broadly applied in a wide range of useful transformations, especially those involving C–C bond formation . This suggests that it will continue to be a valuable tool in chemical synthesis in the future.
Méthodes De Préparation
Synthetic Routes and Reaction Conditions
Samarium(III) iodide is typically prepared by the direct reaction of metallic samarium with iodine. The reaction is as follows :
2 Sm (s) + 3 I₂ (s) → 2 SmI₃ (s)
This reaction is carried out under an inert atmosphere to prevent the oxidation of samarium and the decomposition of the product.
Industrial Production Methods
In industrial settings, the preparation of samarium(III) iodide follows the same synthetic route but on a larger scale. The reaction is conducted in specialized reactors that maintain an inert atmosphere and control the temperature to ensure the complete reaction of samarium with iodine.
Analyse Des Réactions Chimiques
Types of Reactions
Samarium(III) iodide undergoes various types of reactions, including:
Reduction Reactions: Samarium(III) iodide can act as a reducing agent in organic synthesis.
Substitution Reactions: It can participate in nucleophilic substitution reactions, where it facilitates the replacement of a leaving group with a nucleophile.
Elimination Reactions: Samarium(III) iodide can promote elimination reactions, leading to the formation of alkenes from alkyl halides.
Common Reagents and Conditions
Major Products
The major products formed from reactions involving samarium(III) iodide include alcohols, alkenes, and various substituted organic compounds .
Comparaison Avec Des Composés Similaires
Similar Compounds
Samarium(II) iodide (SmI₂):
Europium(III) iodide (EuI₃): Similar to samarium(III) iodide, it is used in organic synthesis and material science for its luminescent properties.
Terbium(III) iodide (TbI₃): Another lanthanide iodide used in luminescent materials and as a catalyst in organic reactions.
Uniqueness
Samarium(III) iodide is unique due to its versatility in promoting a wide range of organic reactions, including reductions, substitutions, and eliminations . Its ability to act as both a reagent and a catalyst makes it a valuable compound in synthetic chemistry and material science .
Propriétés
{ "Design of the Synthesis Pathway": "The synthesis pathway for Samarium(3+);triiodide involves the reaction between Samarium(III) chloride and potassium triiodide in aqueous solution.", "Starting Materials": ["Samarium(III) chloride", "Potassium triiodide", "Water"], "Reaction": [ "Dissolve Samarium(III) chloride in water to form a clear solution", "Dissolve Potassium triiodide in water to form a yellow solution", "Slowly add the potassium triiodide solution to the Samarium(III) chloride solution while stirring", "A brown precipitate of Samarium(III) triiodide will form", "Filter the precipitate and wash with water to remove any impurities", "Dry the Samarium(III) triiodide powder under vacuum at room temperature" ] } | |
Numéro CAS |
13813-25-7 |
Formule moléculaire |
I3Sm |
Poids moléculaire |
531.1 g/mol |
Nom IUPAC |
triiodosamarium |
InChI |
InChI=1S/3HI.Sm/h3*1H;/q;;;+3/p-3 |
Clé InChI |
XQKBFQXWZCFNFF-UHFFFAOYSA-K |
SMILES |
[I-].[I-].[I-].[Sm+3] |
SMILES canonique |
I[Sm](I)I |
13813-25-7 | |
Origine du produit |
United States |
Avertissement et informations sur les produits de recherche in vitro
Veuillez noter que tous les articles et informations sur les produits présentés sur BenchChem sont destinés uniquement à des fins informatives. Les produits disponibles à l'achat sur BenchChem sont spécifiquement conçus pour des études in vitro, qui sont réalisées en dehors des organismes vivants. Les études in vitro, dérivées du terme latin "in verre", impliquent des expériences réalisées dans des environnements de laboratoire contrôlés à l'aide de cellules ou de tissus. Il est important de noter que ces produits ne sont pas classés comme médicaments et n'ont pas reçu l'approbation de la FDA pour la prévention, le traitement ou la guérison de toute condition médicale, affection ou maladie. Nous devons souligner que toute forme d'introduction corporelle de ces produits chez les humains ou les animaux est strictement interdite par la loi. Il est essentiel de respecter ces directives pour assurer la conformité aux normes légales et éthiques en matière de recherche et d'expérimentation.