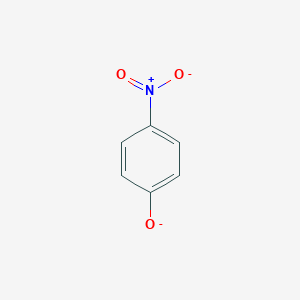
4-Nitrophenolate
Vue d'ensemble
Description
4-Nitrophenolate (C₆H₄NO₃⁻) is the deprotonated form of 4-nitrophenol (4-NP), a weakly acidic compound with a pKa of 7.15 . Under basic conditions (pH > 7.15), 4-NP undergoes deprotonation to form the this compound ion, which exhibits a distinct yellow color and a strong UV-Vis absorption peak at 400 nm . This ion is a critical intermediate in catalytic reduction reactions, particularly in the conversion to 4-aminophenol (4-AP) using reducing agents like NaBH₄ . Its resonance-stabilized structure, facilitated by the para-nitro group, enhances its reactivity and makes it a model substrate for evaluating catalytic performance .
Applications De Recherche Scientifique
Catalytic Applications
4-Nitrophenolate serves as an important intermediate in catalytic processes, especially in the reduction of 4-nitrophenol to 4-aminophenol. This transformation is significant in organic synthesis and pharmaceutical applications.
Green Synthesis of Nanoparticles
Recent studies have highlighted the use of green-synthesized metal nanoparticles (NPs) as catalysts for the reduction of 4-nitrophenol. For instance, gold nanoparticles synthesized using plant extracts have shown remarkable catalytic activity. The following table summarizes various green-synthesized catalysts and their characteristics:
Catalyst Source | Size (nm) | Shape | Reaction Conditions | Rate Constant (K_app) × 10^(-3) s^(-1) |
---|---|---|---|---|
Aspergillus sp. | 4–29 | Spherical | 30 mg L^(-1), 2 mM NaBH₄ | 9.8–25 |
Citrus maxima | 25.7 | Rod/Spherical | 1 mM | NR |
Mung bean starch-AuNPs | 10 | Spherical | 20–100 mg | 0.36 |
These catalysts demonstrate varying efficiencies based on their size, shape, and reaction conditions, showcasing the potential of using biological materials for synthesizing effective catalysts .
Platinum and Palladium Nanoparticles
Platinum and palladium nanoparticles are also effective for reducing 4-nitrophenol. These metals exhibit higher catalytic efficiency compared to other nanoparticles, making them suitable for industrial applications where rapid reaction rates are crucial .
Environmental Remediation
This compound's role in environmental chemistry is significant due to its toxicity and persistence in the environment. Techniques for its detection and reduction are critical for pollution control.
Detection Methods
Innovative detection methods have been developed to identify para-nitrophenol in various environmental samples. These methods include advanced spectroscopic techniques that allow for sensitive and selective identification of this compound in soil and water samples .
Biodegradation Studies
Research has indicated that certain microorganisms can effectively degrade 4-nitrophenol under aerobic conditions. This biodegradation process is essential for mitigating the environmental impact of this compound, particularly in industrial effluents .
Material Science Applications
In material science, this compound derivatives are explored for their optical properties and potential use in nonlinear optical (NLO) applications.
Nonlinear Optical Materials
Studies have shown that organic compounds containing this compound can be utilized to develop NLO materials with desirable properties such as high optical nonlinearity and stability . These materials have applications in telecommunications and photonic devices.
Crystal Growth Studies
The growth of semi-organic crystals incorporating this compound has been investigated, revealing insights into their morphology and potential applications in photonic devices . The control of crystal morphology is crucial for enhancing the performance of these materials.
Analyse Des Réactions Chimiques
a) Enzymatic Oxidation to 4-Nitrocatechol
In Rhodococcus sp. PN1, 4-nitrophenolate undergoes oxidation via a two-component flavin-dependent monooxygenase (NphA1/NphA2):
-
Key Steps :
-
Reaction :
-
Substrate Specificity : The enzyme also oxidizes phenol (kₐₜ = 12 s⁻¹) and 4-chlorophenol (kₐₜ = 8 s⁻¹) .
b) Aqueous Oxidation by Hydroxyl Radicals (- OH)
In atmospheric and aqueous systems, this compound reacts with - OH radicals:
-
Primary Products :
-
Hydroquinone (HQ)
-
1,2,4-Benzenetriol (1,2,4-THB)
-
4-Nitrocatechol (4-NC)
-
-
pH-Dependent Pathways :
Product | Yield (pH 2) | Yield (pH 9) |
---|---|---|
4-Nitrocatechol | 0.11 | 0.25 |
Hydroquinone | 0.05 | 0.06 |
1,2,4-THB | 0.05 | ND |
a) Catalytic Reduction to 4-Aminophenol
This compound is reduced to 4-aminophenol using Au or Ag nanoparticles (NPs) and NaBH₄:
-
Mechanism : Langmuir–Hinshelwood (L–H) model:
-
Adsorption of this compound and BH₄⁻ on the NP surface.
-
Electron transfer from BH₄⁻ to this compound via the catalyst.
-
-
Kinetics :
b) Biological Reduction
In mammals, this compound is metabolized via:
a) Nitrosation with HNO₂
In acidic conditions, this compound reacts with nitrous acid:
-
Primary Product : 2,4-Dinitrophenol.
-
Mechanism :
-
Rate Dependence :
b) Photochemical Nitration
Under UV light, this compound reacts with NO₃⁻/NO₂⁻ to form polynitrated derivatives (e.g., 2,4,6-trinitrophenol) .
Biochemical Transformations
This compound is a substrate for phase I/II metabolism:
-
Phase I :
-
Phase II :
Environmental Degradation
In aquatic systems, this compound resists rapid mineralization:
Q & A
Q. Basic: What experimental methods are recommended for synthesizing 4-nitrophenolate derivatives, and how do pH conditions affect their stability?
This compound is typically synthesized via alkaline deprotonation of 4-nitrophenol (pKa ≈ 7.1). Adjusting pH > 7 ensures complete ionization to the nitrophenolate form, critical for catalytic and spectroscopic studies . For stability, buffer systems (e.g., phosphate or Tris) are recommended to maintain pH during reactions. Kinetic studies using UV-Vis spectroscopy (λ = 400–428 nm) can monitor nitrophenolate degradation or conversion, with extinction coefficients (ε ≈ 18,500 M⁻¹cm⁻¹ at 400 nm) corrected for pH-dependent ionization .
Q. Basic: How can UV-Vis spectroscopy be optimized to quantify this compound in kinetic studies of reduction reactions?
UV-Vis spectroscopy is the standard method for tracking this compound reduction (e.g., to 4-aminophenolate). Key steps:
- Prepare a calibration curve using known concentrations (1–100 µM) at λ = 400 nm.
- Use pseudo-first-order kinetics by maintaining excess NaBH₄ (≥10 mM) to ensure reaction rates depend solely on nitrophenolate concentration .
- Monitor absorbance decay over time and fit data to the Langmuir-Hinshelwood model to calculate rate constants (k) and turnover frequency (TOF). For example, Au/g-Fe₂O₃@HAP catalysts achieved TOF = 241.3 h⁻¹ .
Q. Advanced: How can researchers resolve contradictions in reported catalytic efficiencies for this compound reduction?
Discrepancies in catalytic performance often arise from variations in:
- Synthesis protocols : Catalyst preparation (e.g., deposition–precipitation vs. sol-gel) affects nanoparticle size and dispersion. For Au/g-Fe₂O₃@HAP, smaller Au nanoparticles (<10 nm) enhance surface area and electron transfer .
- Reaction conditions : Temperature, stirring rate, and NaBH₄ concentration influence mass transfer. Standardize conditions (e.g., room temperature, 1500 rpm stirring) for cross-study comparisons.
- Recycling tests : Magnetic separation efficiency and catalyst leaching (e.g., Au loss after 6 cycles) must be quantified via ICP-MS to validate long-term stability claims .
Q. Advanced: What mechanistic insights can be gained from control experiments in this compound hydrolysis studies?
Control experiments are critical to distinguish catalytic vs. non-catalytic pathways:
- Blank reactions : Exclude catalysts to assess spontaneous hydrolysis. For example, zinc(II)-ligand systems showed no activity without this compound, confirming catalysis .
- Isotopic labeling : Use deuterated solvents (D₂O) to probe proton-transfer mechanisms in hydrolysis.
- Spectroscopic trapping : Monitor intermediates via stopped-flow FTIR or NMR to identify transient species (e.g., nitrophenolate–metal complexes) .
Q. Advanced: How do crystallographic techniques improve understanding of this compound-based nonlinear optical (NLO) materials?
Single-crystal X-ray diffraction (SXRD) reveals supramolecular interactions critical for NLO properties. For example:
- 2-Aminopyridinium this compound crystals exhibit charge-transfer interactions between the aromatic amine and nitrophenolate, enhancing hyperpolarizability .
- Thermal analysis : DSC and TGA quantify stability (e.g., melting points >200°C), ensuring suitability for laser applications .
- DFT modeling : Correlate experimental SHG efficiency with theoretical dipole moments to optimize crystal packing .
Q. Advanced: What strategies address reproducibility challenges in synthesizing this compound sensors for anion recognition?
Calix[4]pyrrole-based sensors for nitrophenolate require precise structural modifications:
- Substituent effects : Introduce electron-withdrawing groups (e.g., nitro) to enhance anion binding. A 1999 study achieved a this compound detection limit of 10⁻⁶ M via colorimetric shifts .
- Solvent polarity : Use low-polarity solvents (e.g., CH₂Cl₂) to minimize competition with water for anion binding .
- Competition assays : Test selectivity against common interferents (e.g., Cl⁻, NO₃⁻) using UV-Vis titration .
Q. Methodological: How should researchers design experiments to ensure statistical reliability in this compound data?
- Sample size : Use power analysis to determine replicates (n ≥ 3) for significance (p < 0.05).
- Error bars : Report standard deviation (not SEM) for technical replicates.
- Validation : Cross-check spectroscopic data with HPLC (e.g., for paraoxon hydrolysis byproducts) to confirm this compound quantification accuracy .
Comparaison Avec Des Composés Similaires
4-Nitrophenolate vs. 2-Nitrophenolate
The positional isomerism of the nitro group significantly impacts physicochemical properties:
- Stability and Resonance: this compound exhibits greater resonance stabilization due to the para-nitro group, which delocalizes the negative charge across the aromatic ring more effectively than the ortho-nitro group in 2-nitrophenolate .
- pKa and pH Sensitivity : The pKa of 4-NP (6.90) is lower than that of 2-NP (7.2), leading to earlier deprotonation of 4-NP under mildly basic conditions .
- Sensing Selectivity: Sulfur-doped graphene quantum dots (S-GQDs) show higher selectivity for this compound due to reduced steric hindrance and enhanced resonance stabilization compared to 2-nitrophenolate .
Table 1: Key Differences Between this compound and 2-Nitrophenolate
Property | This compound | 2-Nitrophenolate |
---|---|---|
pKa of Parent Phenol | 6.90 | 7.2 |
UV-Vis λmax | 400 nm | 415 nm (reported elsewhere) |
Resonance Stabilization | High (para-nitro) | Moderate (ortho-nitro) |
This compound vs. 4-Aminophenolate
This compound is a precursor to 4-aminophenolate (C₆H₆NO⁻) via catalytic reduction. Key distinctions include:
- Reduction Kinetics: The reduction of this compound to 4-aminophenolate is highly efficient under catalytic conditions. For example, Au/g-Fe₂O₃@HAP composites achieve a turnover frequency (TOF) of 241.3 h⁻¹ , while Pt/Ni(OH)₂ nanosheets complete the reaction in 150 seconds .
- Mechanistic Pathways: The reduction involves adsorption of this compound onto catalyst surfaces (e.g., Au or Pt nanoparticles), followed by hydride transfer from NaBH₄ . In contrast, 4-aminophenolate is stabilized through interactions with hydroxyl groups on carbon-based catalysts .
Table 2: Catalytic Performance in this compound Reduction
This compound vs. Other Phenolates
- Sulfur-Phenolate Exchange: this compound participates in sulfur(VI) exchange reactions but differs from simpler phenolates (e.g., phenylmethanesulfonate) due to its electron-withdrawing nitro group, which reduces nucleophilicity .
- Adsorption Behavior: The negative charge on this compound causes electrostatic repulsion with negatively charged adsorbents, unlike neutral phenolates, which exhibit higher adsorption capacities .
Role in Catalytic Mechanisms
This compound’s reactivity is influenced by:
- pH-Dependent Speciation: Conversion to this compound begins at pH > 5, with full deprotonation at pH > 7.15 .
- Catalyst Interactions: Au and Pt nanoparticles enhance reduction rates via charge transfer and surface adsorption . For example, AuNPs with hyperbranched polymers leverage electrostatic interactions to accelerate this compound diffusion .
Méthodes De Préparation
Alkaline Hydrolysis of p-Nitrochlorobenzene with Surfactant Mediation
Reaction Mechanism and Process Design
The synthesis of 4-nitrophenolate via alkaline hydrolysis of p-nitrochlorobenzene (p-NCB) follows a nucleophilic aromatic substitution mechanism. Under high-temperature and pressurized conditions, sodium hydroxide displaces the chlorine atom in p-NCB, forming sodium p-nitrophenolate and sodium chloride as a byproduct . The reaction is represented as:
6\text{H}4\text{ClNO}2 + 2\text{NaOH} \rightarrow \text{C}6\text{H}4\text{NO}2\text{ONa} + \text{NaCl} + \text{H}_2\text{O}
A critical advancement in this method involves the addition of quaternary ammonium salt surfactants , which stabilize the reaction interface and prevent localized overheating. For example, a surfactant synthesized from sodium sulfite, epichlorohydrin, and diethylenetriamine reduces aggregation of reactive intermediates, lowering the activation energy by approximately 15% .
Optimized Process Parameters
The Chinese patent CN113200862A details a continuous flow system with the following operational parameters :
Parameter | Optimal Range | Effect on Yield/Purity |
---|---|---|
Reaction Temperature | 160–165°C | Prevents decomposition |
Pressure | 0.75–0.8 MPa | Enhances NaOH reactivity |
p-NCB Flow Rate | 600–5200 g/h | Balances residence time |
NaOH Solution Flow Rate | 5100–5200 g/h | Maintains stoichiometric excess |
Surfactant:p-NCB Ratio | 9:100 (mass) | Minimizes side reactions |
Example 1 : At 162°C and 0.75 MPa, with p-NCB and NaOH flow rates of 620 g/h and 5150 g/h respectively, the yield reaches 94.3% with 99.2% purity. Cooling the reaction mixture to 15°C ensures efficient crystallization .
Hydrolysis-Acidification-Crystallization with Intermediate Concentration
Propriétés
Numéro CAS |
14609-74-6 |
---|---|
Formule moléculaire |
C6H4NO3- |
Poids moléculaire |
138.1 g/mol |
Nom IUPAC |
4-nitrophenolate |
InChI |
InChI=1S/C6H5NO3/c8-6-3-1-5(2-4-6)7(9)10/h1-4,8H/p-1 |
Clé InChI |
BTJIUGUIPKRLHP-UHFFFAOYSA-M |
SMILES |
C1=CC(=CC=C1[N+](=O)[O-])[O-] |
SMILES canonique |
C1=CC(=CC=C1[N+](=O)[O-])[O-] |
Origine du produit |
United States |
Retrosynthesis Analysis
AI-Powered Synthesis Planning: Our tool employs the Template_relevance Pistachio, Template_relevance Bkms_metabolic, Template_relevance Pistachio_ringbreaker, Template_relevance Reaxys, Template_relevance Reaxys_biocatalysis model, leveraging a vast database of chemical reactions to predict feasible synthetic routes.
One-Step Synthesis Focus: Specifically designed for one-step synthesis, it provides concise and direct routes for your target compounds, streamlining the synthesis process.
Accurate Predictions: Utilizing the extensive PISTACHIO, BKMS_METABOLIC, PISTACHIO_RINGBREAKER, REAXYS, REAXYS_BIOCATALYSIS database, our tool offers high-accuracy predictions, reflecting the latest in chemical research and data.
Strategy Settings
Precursor scoring | Relevance Heuristic |
---|---|
Min. plausibility | 0.01 |
Model | Template_relevance |
Template Set | Pistachio/Bkms_metabolic/Pistachio_ringbreaker/Reaxys/Reaxys_biocatalysis |
Top-N result to add to graph | 6 |
Feasible Synthetic Routes
Avertissement et informations sur les produits de recherche in vitro
Veuillez noter que tous les articles et informations sur les produits présentés sur BenchChem sont destinés uniquement à des fins informatives. Les produits disponibles à l'achat sur BenchChem sont spécifiquement conçus pour des études in vitro, qui sont réalisées en dehors des organismes vivants. Les études in vitro, dérivées du terme latin "in verre", impliquent des expériences réalisées dans des environnements de laboratoire contrôlés à l'aide de cellules ou de tissus. Il est important de noter que ces produits ne sont pas classés comme médicaments et n'ont pas reçu l'approbation de la FDA pour la prévention, le traitement ou la guérison de toute condition médicale, affection ou maladie. Nous devons souligner que toute forme d'introduction corporelle de ces produits chez les humains ou les animaux est strictement interdite par la loi. Il est essentiel de respecter ces directives pour assurer la conformité aux normes légales et éthiques en matière de recherche et d'expérimentation.