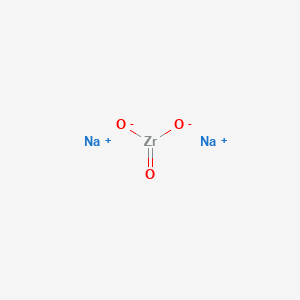
Sodium zirconate
Vue d'ensemble
Description
Sodium zirconate (Na₂ZrO₃) is an inorganic compound synthesized via solid-state reactions, often derived from zircon sand or recycled materials like dental waste . It exhibits a layered perovskite-like structure, contributing to its high thermal stability and reactivity. Key applications include carbon dioxide (CO₂) capture, catalysis in hydrogen production, and use in leaching processes .
Méthodes De Préparation
Synthetic Routes and Reaction Conditions: Sodium zirconate is typically synthesized through the reaction of sodium hydroxide (NaOH) with zirconium dioxide (ZrO₂) at high temperatures. This reaction produces a stable, white crystalline powder . Another method involves the solid-state reaction derived from the thermal decomposition of its precursors, which is analyzed using thermogravimetric analysis techniques .
Industrial Production Methods: In industrial settings, this compound can be produced using a citrate sol-gel method, which allows for synthesis at lower temperatures compared to conventional solid-state methods. This method involves the use of citric acid as a chelating agent, resulting in this compound with excellent carbon dioxide adsorption properties .
Analyse Des Réactions Chimiques
Thermal Decomposition of Precursors
A novel mechanism involves the reaction of sodium acetate (CH₃COONa) and zirconium(IV) acetylacetonate (Zr(C₅H₇O₂)₄) in ethanol. The process occurs in three stages :
Reaction Step | Temperature Range | Chemical Equation | Byproducts |
---|---|---|---|
1. Decomposition of Zr(C₅H₇O₂)₄ | 190–340°C | CO, H₂O, acetone | |
2. Sodium Acetate Decomposition | 400–550°C | CO₂, acetone | |
3. Final Solid-State Reaction | >550°C | CO₂ |
This pathway produces 99% pure Na₂ZrO₃ confirmed via X-ray diffraction (XRD) .
Alkaline Fusion Method
An alternative synthesis involves reacting zirconium silicate (ZrSiO₄) with sodium hydroxide at >600°C :
This method yields a mixture of this compound, sodium silicate, and sodium zirconium silicate, requiring subsequent purification .
Kinetic Analysis of Formation
The Arrhenius equation models the thermal decomposition kinetics. Key parameters derived from thermogravimetric (TG) data include :
Parameter | Stage 1 | Stage 2 | Stage 3 |
---|---|---|---|
Activation Energy (Eₐ) | 98 kJ/mol | 120 kJ/mol | 145 kJ/mol |
Pre-exponential Factor (A) | |||
Reaction Order (n) | 0.7 | 1.0 | 1.2 |
At higher temperatures (>700°C), decomposition rates increase significantly, reducing Na₂ZrO₃ formation time by ~40% .
Hydrolysis and Acid Reactions
This compound reacts with water and acids, influencing its stability and applications:
-
Hydrolysis :
This reaction is critical in ceramic pigment production, where controlled hydrolysis prevents phase instability .
-
Sulfuric Acid Reaction :
Used industrially to produce zirconium sulfate for tanning and coatings .
High-Temperature Stability
Na₂ZrO₃ exhibits robust thermal stability, melting at 851°C and maintaining structural integrity up to 1200°C. Its density (4.74 g/cm³) and refractory properties make it suitable for aerospace and nuclear applications .
Applications De Recherche Scientifique
Carbon Dioxide Capture
Sodium zirconate is primarily recognized for its effectiveness as a high-temperature carbon dioxide (CO₂) sorbent. Its unique properties make it suitable for capturing CO₂ in industrial processes, such as steel and cement manufacturing.
Performance Characteristics
- Sorption Capacity : this compound exhibits a high CO₂ adsorption capacity, which can vary based on synthesis methods and conditions. For instance, research indicates that this compound synthesized through spray drying can achieve approximately 75% of its theoretical capacity within just 5 minutes of exposure to CO₂ at 700 °C .
- Stability : The material demonstrates stable multicycle performance, essential for practical applications in cyclic processes .
Case Studies
- Research by Munro et al. (2020) : This study explored various solid-state synthesis methods, concluding that ball milling could optimize CO₂ uptake due to improved mineral phase purity and crystal size .
- Bamiduro et al. (2024) : Investigated the impact of synthesis heating rates on CO₂ carbonation and desorption, revealing that slower heating rates lead to higher purity and better performance in CO₂ uptake .
Catalysis
This compound also serves as a solid base catalyst in biodiesel production. Its catalytic properties are particularly effective in transesterification reactions.
Catalytic Activity
- Transesterification of Oils : this compound has been utilized as a catalyst for converting vegetable oils into biodiesel, demonstrating effective performance when combined with various feedstocks such as soybean and Jatropha curcas oil.
Feedstock | Catalyst Used | Reaction Conditions | Yield (%) |
---|---|---|---|
Soybean Oil | Na₂ZrO₃ | 60 °C, 3 hours | 95 |
Jatropha Curcas Oil | Na₂ZrO₃ | 65 °C, 4 hours | 90 |
Research Findings
- A study conducted by Zubair et al. (2017) highlighted the effect of calcination temperature on the catalytic efficiency of this compound, showing that optimal conditions significantly enhance biodiesel yield .
Nuclear Waste Management
Another critical application of this compound is in the immobilization of radioactive waste. Its ability to form stable compounds with radionuclides makes it valuable for safe disposal methods.
Mécanisme D'action
The mechanism by which sodium zirconate exerts its effects is primarily through its ability to act as a carbon dioxide sorbent. It captures carbon dioxide through a chemisorption process, where carbon dioxide molecules react with this compound to form sodium carbonate and zirconium dioxide. This process is facilitated by the mobility of sodium ions within the this compound structure, allowing for efficient carbon dioxide capture .
Comparaison Avec Des Composés Similaires
Industrial Relevance :
- Patented by CIMAV (Mexico) for its cost-effectiveness, durability, and superior CO₂ capture performance compared to Japanese synthetic and natural materials .
- Enhances hydrogen yield in biomass pyrolysis by sequestering CO₂ in situ, promoting cleaner energy production .
Comparison with Similar Zirconate Compounds
Sodium Zirconate vs. Lithium Zirconate (Li₂ZrO₃)
Key Findings :
- This compound outperforms lithium zirconate in cost and scalability due to its use of waste-derived precursors .
- Lithium zirconate, however, is more studied for high-temperature CO₂ capture in energy systems .
This compound vs. Gadolinium Zirconate (Gd₂Zr₂O₇)
Key Findings :
- Gadolinium zirconate is preferred in TBCs for its low thermal conductivity and phase stability, whereas this compound excels in gas-separation applications .
- This compound’s chemical stability in CO₂-rich environments contrasts with gadolinium zirconate’s vulnerability to vanadium-based corrosion .
This compound vs. Barium Zirconate (BaZrO₃)
Key Findings :
- Barium zirconate is critical in SOFCs due to its proton conductivity, while this compound lacks ionic transport utility .
- This compound’s exothermic leaching behavior contrasts with barium zirconate’s endothermic sintering requirements .
This compound vs. Sodium Zirconium Silicate (Na₂ZrSiO₅)
Key Findings :
- Synthesis conditions (NaOH ratio) dictate the final product, with this compound favoring alkaline environments .
- This compound’s hydrolytic reactivity enables CO₂ capture, unlike the inert silicate variant .
Table 1: Comparative Performance in CO₂ Capture
Compound | CO₂ Uptake (g/g) | Cyclability (cycles) | Cost Efficiency | Reference |
---|---|---|---|---|
This compound | 0.195 | 30 | High | |
Lithium Zirconate | 0.18–0.20 | 20–25 | Moderate |
Table 2: Leaching Kinetics in HCl
Compound | Optimal Temp (°C) | Conversion (%) | Activation Energy (kJ/mol) | Reference |
---|---|---|---|---|
This compound | 50 | 43.43 | -62.974 |
Activité Biologique
Sodium zirconate (Na2ZrO3) is a compound that has gained attention for its potential applications in various fields, particularly in biomedicine. This article explores the biological activity of this compound, focusing on its mechanisms, efficacy in medical applications, and relevant research findings.
Overview of this compound
This compound is an inorganic compound with properties that make it suitable for use in catalysis and as a potential therapeutic agent. Its unique structure allows it to interact with biological systems, which has led to investigations into its biocompatibility and therapeutic potential.
This compound exhibits several mechanisms of action that contribute to its biological activity:
- Ion Exchange Properties : this compound functions as a potassium binder, similar to other cation exchange resins. It selectively binds potassium ions in the gastrointestinal tract, facilitating their excretion and thereby lowering serum potassium levels in conditions like hyperkalemia .
- Biocompatibility : Studies have shown that this compound coatings on stainless steel substrates demonstrate excellent corrosion resistance and biocompatibility, making them suitable for biomedical applications such as implants .
Hyperkalemia Treatment
Recent clinical trials have evaluated the efficacy of sodium zirconium cyclosilicate (SZC), a related compound, in treating hyperkalemia. SZC has been shown to significantly reduce serum potassium levels:
- Clinical Trial Findings : In a multicenter study involving patients with elevated serum potassium levels, SZC demonstrated a mean reduction of 0.41 mmol/L compared to placebo over four hours . The rapid onset of action (within one hour) highlights its potential as an adjunct therapy during hyperkalemic emergencies.
- Comparison with Other Treatments : SZC's selectivity for potassium allows it to bind potassium ions effectively in the upper gastrointestinal tract, unlike traditional resins that primarily act in the colon .
Case Studies
- Pilot Study on Emergency Treatment : A pilot evaluation explored the use of SZC alongside insulin and glucose for emergency treatment of hyperkalemia. The study found that patients receiving SZC required fewer additional potassium-lowering therapies compared to those on placebo .
- Corrosion Resistance Studies : Research on this compound coatings indicated significant improvements in corrosion resistance when applied to 316L stainless steel, suggesting its potential use in long-term biomedical implants .
Table 1: Summary of Key Studies on this compound
Q & A
Basic Research Questions
Q. What are the primary laboratory synthesis methods for sodium zirconate, and how do thermodynamic calculations guide parameter selection?
this compound is commonly synthesized via solid-state reactions or caustic fusion of zircon sand (ZrSiO₄) with NaOH. Thermodynamic modeling using software like HSC helps predict reaction pathways and equilibrium phases. For example, in ZrSiO₄-NaOH-CaO systems, this compound forms stably at 600–900°C, with NaOH ratios and temperature dictating silicate byproduct formation . Solid-state synthesis involves calcining stoichiometric mixtures of Na₂CO₃ and ZrO₂ at ~900°C, validated by XRD to confirm phase purity .
Q. How is this compound structurally characterized to confirm phase purity and crystallinity?
X-ray diffraction (XRD) is the primary method for phase identification, distinguishing this compound from intermediates like Na₂ZrSiO₅. Solid-state nuclear magnetic resonance (NMR) and electron microscopy (HRTEM) are used to probe local atomic environments and crystallite morphology. For instance, Al-doped Na₂ZrO₃ samples show aluminum occupancy in Zr or Na sites, detected via ²⁷Al NMR .
Q. What role does this compound play in enhancing hydrogen production during biomass pyrolysis, and how is its CO₂ capture capacity quantified?
this compound acts as a CO₂ sorbent during pyrolysis, shifting equilibrium to favor H₂ production via Le Chatelier’s principle. Its capacity is measured thermogravimetrically, achieving 0.195 g CO₂/g sorbent over 30 cycles (82% theoretical uptake). This cyclic stability is attributed to reversible carbonation (Na₂ZrO₃ + CO₂ → Na₂CO₃ + ZrO₂) .
Advanced Research Questions
Q. How can CO₂ chemisorption in this compound be optimized through doping, and what techniques assess structural modifications?
Aluminum doping (Na₂(Zr₁₋ₓAlₓ)O₃) enhances CO₂ uptake by creating defects that improve ion mobility. Optimal doping (e.g., x = 0.1) increases uptake by 20% compared to pure Na₂ZrO₃. Kinetic analyses using Arrhenius plots reveal lowered activation enthalpies (~50 kJ/mol) due to facilitated Na⁺ diffusion. Post-capture XRD identifies secondary phases (e.g., NaAlO₂), which influence stability .
Q. What kinetic models describe this compound leaching in HCl, and how are activation energy and optimal conditions determined?
The shrinking core model (chemical reaction-controlled) is applied, with variables like temperature (50–90°C), HCl:feed ratio (5–9 mol), and time (10–50 min). Rate constants (e.g., 0.0027 min⁻¹ at 50°C) are derived from linearized plots of vs. time. Activation energy () is calculated via the Arrhenius equation, though anomalous negative values (e.g., −62.974 kJ/mol in one study) suggest complex interfacial dynamics .
Q. How do contradictions in reported synthesis conditions for this compound arise, and how can experimental design resolve them?
Discrepancies in optimal temperatures (e.g., 650°C vs. 900°C) stem from differences in reactant ratios and heating rates. For instance, NaOH excess lowers melting points, enabling lower-temperature synthesis. Controlled non-isothermal studies (TG-DTA) identify reaction thresholds, while iterative XRD during synthesis monitors phase evolution to refine protocols .
Q. What mechanisms explain this compound’s stability in corrosive environments, and how do sulfate vs. chloride salts affect degradation?
In thermal barrier coatings, Na₂ZrO₃ resists sulfate corrosion but degrades in chloride-rich environments due to bond coat (MCrAlY) attack. Multilayer coatings with YSZ (yttria-stabilized zirconia) improve durability, as YSZ blocks salt penetration. Post-exposure SEM/EDS reveals ZrO₂-rich passivation layers that mitigate spallation .
Q. Methodological Considerations
- Experimental Design : When optimizing doping or synthesis, use fractional factorial designs to test multiple variables (e.g., doping level, temperature, dwell time) efficiently .
- Data Contradictions : Address discrepancies in activation energies by standardizing leaching protocols (e.g., particle size, agitation) and validating models with in situ Raman spectroscopy .
- Cyclic Testing : For CO₂ capture studies, employ thermogravimetric analysis (TGA) with controlled gas flow rates to ensure reproducibility across cycles .
Propriétés
IUPAC Name |
disodium;dioxido(oxo)zirconium | |
---|---|---|
Source | PubChem | |
URL | https://pubchem.ncbi.nlm.nih.gov | |
Description | Data deposited in or computed by PubChem | |
InChI |
InChI=1S/2Na.3O.Zr/q2*+1;;2*-1; | |
Source | PubChem | |
URL | https://pubchem.ncbi.nlm.nih.gov | |
Description | Data deposited in or computed by PubChem | |
InChI Key |
FQTDUMBPDRMZJF-UHFFFAOYSA-N | |
Source | PubChem | |
URL | https://pubchem.ncbi.nlm.nih.gov | |
Description | Data deposited in or computed by PubChem | |
Canonical SMILES |
[O-][Zr](=O)[O-].[Na+].[Na+] | |
Source | PubChem | |
URL | https://pubchem.ncbi.nlm.nih.gov | |
Description | Data deposited in or computed by PubChem | |
Molecular Formula |
Na2O3Zr | |
Source | PubChem | |
URL | https://pubchem.ncbi.nlm.nih.gov | |
Description | Data deposited in or computed by PubChem | |
Molecular Weight |
185.20 g/mol | |
Source | PubChem | |
URL | https://pubchem.ncbi.nlm.nih.gov | |
Description | Data deposited in or computed by PubChem | |
Physical Description |
Off-white odorless powder; [Alfa Aesar MSDS] | |
Record name | Sodium zirconate | |
Source | Haz-Map, Information on Hazardous Chemicals and Occupational Diseases | |
URL | https://haz-map.com/Agents/17680 | |
Description | Haz-Map® is an occupational health database designed for health and safety professionals and for consumers seeking information about the adverse effects of workplace exposures to chemical and biological agents. | |
Explanation | Copyright (c) 2022 Haz-Map(R). All rights reserved. Unless otherwise indicated, all materials from Haz-Map are copyrighted by Haz-Map(R). No part of these materials, either text or image may be used for any purpose other than for personal use. Therefore, reproduction, modification, storage in a retrieval system or retransmission, in any form or by any means, electronic, mechanical or otherwise, for reasons other than personal use, is strictly prohibited without prior written permission. | |
CAS No. |
12201-48-8 | |
Record name | Zirconate (ZrO32-), sodium (1:2) | |
Source | EPA Chemicals under the TSCA | |
URL | https://www.epa.gov/chemicals-under-tsca | |
Description | EPA Chemicals under the Toxic Substances Control Act (TSCA) collection contains information on chemicals and their regulations under TSCA, including non-confidential content from the TSCA Chemical Substance Inventory and Chemical Data Reporting. | |
Record name | Disodium zirconate | |
Source | European Chemicals Agency (ECHA) | |
URL | https://echa.europa.eu/substance-information/-/substanceinfo/100.032.150 | |
Description | The European Chemicals Agency (ECHA) is an agency of the European Union which is the driving force among regulatory authorities in implementing the EU's groundbreaking chemicals legislation for the benefit of human health and the environment as well as for innovation and competitiveness. | |
Explanation | Use of the information, documents and data from the ECHA website is subject to the terms and conditions of this Legal Notice, and subject to other binding limitations provided for under applicable law, the information, documents and data made available on the ECHA website may be reproduced, distributed and/or used, totally or in part, for non-commercial purposes provided that ECHA is acknowledged as the source: "Source: European Chemicals Agency, http://echa.europa.eu/". Such acknowledgement must be included in each copy of the material. ECHA permits and encourages organisations and individuals to create links to the ECHA website under the following cumulative conditions: Links can only be made to webpages that provide a link to the Legal Notice page. | |
Avertissement et informations sur les produits de recherche in vitro
Veuillez noter que tous les articles et informations sur les produits présentés sur BenchChem sont destinés uniquement à des fins informatives. Les produits disponibles à l'achat sur BenchChem sont spécifiquement conçus pour des études in vitro, qui sont réalisées en dehors des organismes vivants. Les études in vitro, dérivées du terme latin "in verre", impliquent des expériences réalisées dans des environnements de laboratoire contrôlés à l'aide de cellules ou de tissus. Il est important de noter que ces produits ne sont pas classés comme médicaments et n'ont pas reçu l'approbation de la FDA pour la prévention, le traitement ou la guérison de toute condition médicale, affection ou maladie. Nous devons souligner que toute forme d'introduction corporelle de ces produits chez les humains ou les animaux est strictement interdite par la loi. Il est essentiel de respecter ces directives pour assurer la conformité aux normes légales et éthiques en matière de recherche et d'expérimentation.