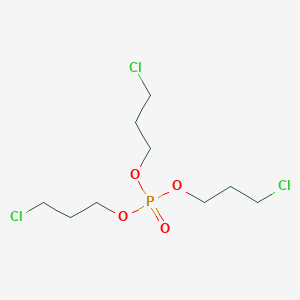
Tris(3-chloropropyl)phosphate
Vue d'ensemble
Description
Tris(3-chloropropyl) phosphate is a chlorinated organophosphate compound widely used as a flame retardant. It is commonly added to polyurethane foams, polyvinyl chloride (PVC), and ethylene-vinyl acetate (EVA) to enhance their fire resistance. The compound is known for its effectiveness in reducing the flammability of materials, making it a valuable additive in various industrial applications.
Applications De Recherche Scientifique
Tris(3-chloropropyl) phosphate has a wide range of scientific research applications:
Chemistry: It is used as a flame retardant in the synthesis of polymers and other materials.
Medicine: Research is ongoing to understand its potential health effects and mechanisms of action.
Mécanisme D'action
Target of Action
Tris(3-chloropropyl)phosphate (TCPP) is a chlorinated organophosphate flame retardant . It is commonly added to polyurethane foams and minor amounts are used in PVC and EVA . The primary targets of TCPP are these materials, where it acts as a flame retardant.
Mode of Action
The mode of action of TCPP is primarily physical rather than chemical. As a flame retardant, TCPP works by inhibiting the combustion process. It does this by forming a protective layer on the material’s surface, which prevents oxygen from reaching the material and fueling the fire .
Biochemical Pathways
Research indicates that tcpp can cause disturbances in cell growth/division, gene expression, energy and material metabolism, signal transduction, defense, and cytoskeleton .
Result of Action
The primary result of TCPP’s action is its flame-retardant properties. By inhibiting the combustion process, TCPP can significantly slow the spread of fire, providing crucial time for individuals to escape and for firefighters to respond . On a molecular and cellular level, TCPP has been shown to cause disturbances in various cellular processes, as mentioned above .
Action Environment
The action of TCPP can be influenced by various environmental factors. For example, the effectiveness of TCPP as a flame retardant can be affected by the temperature and the presence of other combustible materials. Additionally, TCPP can be released into the environment through volatilization, abrasion, and dissolution, which can be influenced by factors such as temperature, humidity, and the presence of other chemicals .
Analyse Biochimique
Biochemical Properties
It is known that Tris(3-chloropropyl)phosphate is comprised of four isomers . These isomers can potentially interact with different enzymes, proteins, and other biomolecules, affecting their function and potentially leading to various biochemical reactions .
Cellular Effects
This compound can have various effects on cells and cellular processes. For example, exposure to this compound has been implicated in hepatotoxicity . It can also cause DNA damage and cell cycle arrest in GC-2 cells, and ultimately affect the growth and development of spermatocytes .
Molecular Mechanism
It is known that this compound can cause DNA damage . This suggests that it may interact with DNA and other biomolecules at the molecular level, potentially leading to changes in gene expression .
Temporal Effects in Laboratory Settings
The effects of this compound can change over time in laboratory settings. For example, exposure to this compound can induce marginally significant micronuclei (MN) frequencies as well as cytotoxic effects .
Dosage Effects in Animal Models
The effects of this compound can vary with different dosages in animal models. For example, treatment with 30 and 40 μg mL −1 of this compound induced marginally significant micronuclei (MN) frequencies as well as cytotoxic effects .
Metabolic Pathways
It is known that this compound can cause disturbances in fat metabolism and liver integrity .
Subcellular Localization
It is known that this compound can cause DNA damage , suggesting that it may localize to the nucleus where it interacts with DNA.
Méthodes De Préparation
Synthetic Routes and Reaction Conditions
Tris(3-chloropropyl) phosphate is synthesized through the reaction of propylene oxide with phosphoryl chloride. This reaction typically produces a mixture of isomers, with tris(2-chloro-1-methylethyl) phosphate being the most dominant isomer . The reaction conditions involve maintaining a controlled temperature and pressure to ensure the desired product yield.
Industrial Production Methods
In industrial settings, tris(3-chloropropyl)phosphate is produced by reacting propylene oxide with phosphoryl chloride in large-scale reactors. The process involves careful monitoring of reaction parameters to optimize the yield and purity of the product. The resulting mixture is then subjected to purification processes to isolate the desired isomer.
Analyse Des Réactions Chimiques
Types of Reactions
Tris(3-chloropropyl) phosphate undergoes various chemical reactions, including:
Oxidation: The compound can be oxidized under specific conditions to form different oxidation products.
Reduction: Reduction reactions can modify the phosphate group, leading to the formation of reduced derivatives.
Substitution: The chlorine atoms in the compound can be substituted with other functional groups through nucleophilic substitution reactions.
Common Reagents and Conditions
Oxidation: Common oxidizing agents include hydrogen peroxide and potassium permanganate.
Reduction: Reducing agents such as lithium aluminum hydride can be used.
Substitution: Nucleophiles like hydroxide ions or amines are commonly employed in substitution reactions.
Major Products Formed
The major products formed from these reactions depend on the specific reagents and conditions used. For example, oxidation may yield phosphoric acid derivatives, while substitution reactions can produce various substituted phosphates.
Comparaison Avec Des Composés Similaires
Similar Compounds
- Tris(2-chloroethyl)phosphate
- Tris(2-chloroisopropyl)phosphate
- Tris(1-chloro-2-propyl)phosphate
Uniqueness
Tris(3-chloropropyl) phosphate is unique due to its specific isomeric structure, which provides distinct flame-retardant properties compared to other similar compounds. Its effectiveness in various applications, particularly in polyurethane foams, sets it apart from other organophosphate flame retardants .
Propriétés
IUPAC Name |
tris(3-chloropropyl) phosphate | |
---|---|---|
Source | PubChem | |
URL | https://pubchem.ncbi.nlm.nih.gov | |
Description | Data deposited in or computed by PubChem | |
InChI |
InChI=1S/C9H18Cl3O4P/c10-4-1-7-14-17(13,15-8-2-5-11)16-9-3-6-12/h1-9H2 | |
Source | PubChem | |
URL | https://pubchem.ncbi.nlm.nih.gov | |
Description | Data deposited in or computed by PubChem | |
InChI Key |
WOURXYYHORRGQO-UHFFFAOYSA-N | |
Source | PubChem | |
URL | https://pubchem.ncbi.nlm.nih.gov | |
Description | Data deposited in or computed by PubChem | |
Canonical SMILES |
C(COP(=O)(OCCCCl)OCCCCl)CCl | |
Source | PubChem | |
URL | https://pubchem.ncbi.nlm.nih.gov | |
Description | Data deposited in or computed by PubChem | |
Molecular Formula |
C9H18Cl3O4P | |
Source | PubChem | |
URL | https://pubchem.ncbi.nlm.nih.gov | |
Description | Data deposited in or computed by PubChem | |
DSSTOX Substance ID |
DTXSID80862522 | |
Record name | Tris(3-chloropropyl)phosphate | |
Source | EPA DSSTox | |
URL | https://comptox.epa.gov/dashboard/DTXSID80862522 | |
Description | DSSTox provides a high quality public chemistry resource for supporting improved predictive toxicology. | |
Molecular Weight |
327.6 g/mol | |
Source | PubChem | |
URL | https://pubchem.ncbi.nlm.nih.gov | |
Description | Data deposited in or computed by PubChem | |
CAS No. |
1067-98-7, 26248-87-3 | |
Record name | 1-Propanol, 3-chloro-, phosphate (3:1) | |
Source | ChemIDplus | |
URL | https://pubchem.ncbi.nlm.nih.gov/substance/?source=chemidplus&sourceid=0001067987 | |
Description | ChemIDplus is a free, web search system that provides access to the structure and nomenclature authority files used for the identification of chemical substances cited in National Library of Medicine (NLM) databases, including the TOXNET system. | |
Record name | 1-Propanol, 2-chloro-, phosphate (3:1), mixed with 1-chloro-2-propanol phosphate (3:1) | |
Source | ChemIDplus | |
URL | https://pubchem.ncbi.nlm.nih.gov/substance/?source=chemidplus&sourceid=0026248873 | |
Description | ChemIDplus is a free, web search system that provides access to the structure and nomenclature authority files used for the identification of chemical substances cited in National Library of Medicine (NLM) databases, including the TOXNET system. | |
Record name | Tris(3-chloropropyl)phosphate | |
Source | EPA DSSTox | |
URL | https://comptox.epa.gov/dashboard/DTXSID80862522 | |
Description | DSSTox provides a high quality public chemistry resource for supporting improved predictive toxicology. | |
Retrosynthesis Analysis
AI-Powered Synthesis Planning: Our tool employs the Template_relevance Pistachio, Template_relevance Bkms_metabolic, Template_relevance Pistachio_ringbreaker, Template_relevance Reaxys, Template_relevance Reaxys_biocatalysis model, leveraging a vast database of chemical reactions to predict feasible synthetic routes.
One-Step Synthesis Focus: Specifically designed for one-step synthesis, it provides concise and direct routes for your target compounds, streamlining the synthesis process.
Accurate Predictions: Utilizing the extensive PISTACHIO, BKMS_METABOLIC, PISTACHIO_RINGBREAKER, REAXYS, REAXYS_BIOCATALYSIS database, our tool offers high-accuracy predictions, reflecting the latest in chemical research and data.
Strategy Settings
Precursor scoring | Relevance Heuristic |
---|---|
Min. plausibility | 0.01 |
Model | Template_relevance |
Template Set | Pistachio/Bkms_metabolic/Pistachio_ringbreaker/Reaxys/Reaxys_biocatalysis |
Top-N result to add to graph | 6 |
Feasible Synthetic Routes
Q1: What can you tell me about the environmental presence of Tris(3-chloropropyl)phosphate based on this study?
A1: The study identified this compound as one of the most abundant and widespread contaminants across the 32 surface water sampling sites investigated []. This suggests that this compound is entering the aquatic environment, potentially through various pathways such as industrial discharge or agricultural runoff. The study emphasizes the importance of monitoring programs like this to better understand the presence and potential risks of such compounds in water resources.
Q2: The study doesn't focus on the toxicity of this compound. Why is its presence in surface water concerning?
A2: While this specific research didn't delve into toxicological effects, the widespread presence of this compound raises concerns due to its classification as a potential carcinogen and its persistence in the environment []. Further research is crucial to determine the potential long-term ecological and human health impacts of this compound and other emerging contaminants found in surface waters.
Avertissement et informations sur les produits de recherche in vitro
Veuillez noter que tous les articles et informations sur les produits présentés sur BenchChem sont destinés uniquement à des fins informatives. Les produits disponibles à l'achat sur BenchChem sont spécifiquement conçus pour des études in vitro, qui sont réalisées en dehors des organismes vivants. Les études in vitro, dérivées du terme latin "in verre", impliquent des expériences réalisées dans des environnements de laboratoire contrôlés à l'aide de cellules ou de tissus. Il est important de noter que ces produits ne sont pas classés comme médicaments et n'ont pas reçu l'approbation de la FDA pour la prévention, le traitement ou la guérison de toute condition médicale, affection ou maladie. Nous devons souligner que toute forme d'introduction corporelle de ces produits chez les humains ou les animaux est strictement interdite par la loi. Il est essentiel de respecter ces directives pour assurer la conformité aux normes légales et éthiques en matière de recherche et d'expérimentation.