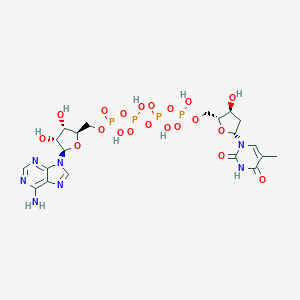
P(1)-(Adenosine-5')-P(5)-(thymidine-5')-pentaphosphate
Vue d'ensemble
Description
P(1)-(Adenosine-5')-P(5)-(Thymidine-5')-pentaphosphate (hereafter referred to by its full name) is a dinucleoside pentaphosphate compound comprising adenosine and thymidine moieties linked by a pentaphosphate bridge. It has been identified as a structural analogue of natural nucleotide polyphosphates, with applications in enzymology and antimicrobial research. For instance, it was utilized in crystallographic studies of Mycobacterium tuberculosis thymidylate kinase (mtTMPK), where its removal from the enzyme's crystal structure (PDB ID: 1MRN) facilitated inhibitor screening .
This compound’s unique adenosine-thymidine pairing distinguishes it from more common dinucleoside polyphosphates, such as diadenosine pentaphosphate (Ap5A), which feature two adenosine groups. Its synthesis likely involves specialized condensation methods, as thymidine derivatives often require protection of exocyclic amino groups during phosphorylation, a challenge noted in analogous triphosphate syntheses .
Activité Biologique
P(1)-(Adenosine-5')-P(5)-(thymidine-5')-pentaphosphate, commonly referred to as Ap5A, is a significant diadenosine polyphosphate with a variety of biological activities. This compound is characterized by its unique structure, which consists of two adenosine molecules linked by a pentaphosphate chain. Its biological roles are primarily related to its function as an inhibitor of adenylate kinase and its interactions with various cellular processes.
Ap5A has the molecular formula and is often synthesized through a two-step process involving adenosine 5'-tetraphosphate (P4A) and trimetaphosphate (P3) . The synthesis requires specific pH conditions (7.5 to 8.5) and is influenced by metal ions, which are critical for its stability and activity.
1. Inhibition of Adenylate Kinase
Ap5A is recognized as a potent inhibitor of adenylate kinase, an enzyme that plays a crucial role in cellular energy metabolism by facilitating the conversion between ATP and ADP. In studies involving fragmented sarcoplasmic reticulum from bullfrog skeletal muscle, Ap5A demonstrated competitive inhibition of adenylate kinase activity without affecting calcium uptake or ATPase activity . This property makes Ap5A a valuable tool in biochemical experiments aimed at understanding ATP-related processes.
2. Modulation of Calcium Channels
In cardiac muscle cells, Ap5A has been shown to significantly increase the open probability of ryanodine receptor (RyR2) gates at low concentrations (pM to nM). This effect results in prolonged calcium release due to slow dissociation from the receptor, indicating that Ap5A may play a role in calcium signaling pathways critical for cardiac function .
3. Interaction with Thymidine Kinase
Research has indicated that Ap5A can act as an inhibitor of thymidine kinase, particularly in the context of acute myelocytic leukemia. The compound exhibited varying Ki values depending on the length of the polyphosphate chain, with Ap5A showing effective inhibition at concentrations around 0.12 µM . This suggests potential therapeutic applications in targeting cancer cell metabolism.
Case Studies
Case Study 1: Adenylate Kinase Inhibition
In a controlled experiment, Ap5A was utilized to inhibit adenylate kinase in fragmented sarcoplasmic reticulum preparations. The results showed that concentrations above 50 µM were necessary for complete inhibition, allowing researchers to isolate ATP-related reactions without interference from adenylate kinase activity .
Case Study 2: Cardiac Function Modulation
In cardiac myocytes, low concentrations of Ap5A enhanced calcium release via RyR2 channels, demonstrating its potential as a modulator in cardiac signaling pathways. This effect was observed to be sustained over time due to the compound's slow dissociation from the receptor .
Summary Table of Biological Activities
Biological Activity | Effect | Concentration |
---|---|---|
Inhibition of Adenylate Kinase | Competitive inhibition | >50 µM |
Modulation of RyR2 | Increased open probability | pM to nM |
Inhibition of Thymidine Kinase | Effective inhibitor | 0.12 µM |
Applications De Recherche Scientifique
Enzymatic Inhibition
Ap5A is primarily known for its role as an adenylate kinase inhibitor , which has implications in various biochemical assays and studies.
- Adenylate Kinase Inhibition : Ap5A acts as a potent multisubstrate inhibitor of adenylate kinase, which catalyzes the conversion of ATP and AMP into two ADPs. The compound's ability to inhibit this enzyme has been utilized in studies examining the regulation of ATP levels in different cell types. For instance, research indicates that Ap5A effectively inhibits adenylate kinase activity in fragmented sarcoplasmic reticulum from bullfrog skeletal muscle without affecting calcium uptake activities .
Cellular Signaling
Ap5A plays a significant role in cellular signaling pathways, particularly those involving calcium dynamics and muscle contraction.
- Calcium Release Modulation : In cardiac muscle cells, low concentrations of Ap5A can significantly increase the open probability of ryanodine receptor gates, which are critical for calcium release during muscle contraction. This effect is attributed to the slow dissociation of Ap5A from the receptor, prolonging its action .
Research Tool in Biochemical Studies
Due to its specific inhibitory properties, Ap5A has become a valuable tool in various experimental setups.
- Experimental Control : Researchers have used Ap5A to avoid complications arising from endogenous adenylate kinase activity in experiments involving calcium uptake and ATP-ADP exchange reactions. This application helps clarify the roles of other enzymes and substrates in cellular processes .
Diagnostic Applications
Ap5A also finds utility in diagnostic reagents, particularly in assays measuring enzyme activities.
- Creatine Kinase Determination : The compound has been employed in diagnostic reagents to inhibit adenylate kinase during the determination of creatine kinase activity. This inhibition is crucial for accurate measurements in clinical settings .
Case Study 1: Adenylate Kinase Inhibition
In a study examining the effects of Ap5A on fragmented sarcoplasmic reticulum, it was found that concentrations above 50 µM were necessary for complete inhibition of adenylate kinase. This finding underscores the importance of concentration-dependent effects when using Ap5A as an experimental tool .
Case Study 2: Calcium Dynamics
Research demonstrated that Ap5A significantly enhances calcium release from ryanodine receptors in cardiac muscle at picomolar to nanomolar concentrations. This property is being explored for potential therapeutic applications in cardiac dysfunctions where calcium signaling is disrupted .
Q & A
Q. What is the structural configuration of P(1)-(Adenosine-5')-P(5)-(Thymidine-5')-pentaphosphate, and how does it enable bisubstrate enzyme inhibition?
Basic
The compound consists of adenosine and thymidine moieties linked via a pentaphosphate bridge (A(5')-P5-P5'-T(5')). The adenosine and thymidine are esterified to the terminal phosphate groups of the pentaphosphate chain, creating a linear bisubstrate analog. This configuration mimics the transition state of enzymes that process both adenosine and thymidine nucleotides, such as thymidylate kinase or adenylate kinase, by occupying both substrate-binding sites simultaneously . Structural studies (e.g., X-ray crystallography) reveal that the pentaphosphate backbone facilitates high-affinity binding through electrostatic interactions with conserved lysine/arginine residues in enzyme active sites .
Q. What methodologies are recommended for synthesizing and purifying this compound?
Basic
Synthesis typically involves coupling adenosine-5'-monophosphate (AMP) and thymidine-5'-monophosphate (TMP) via a pentaphosphate linker using phosphoramidite or enzymatic ligation strategies. Post-synthesis, purification is achieved via reverse-phase HPLC (≥95% purity) with ion-pairing agents (e.g., triethylammonium acetate) to resolve charged species. Sodium or lithium salt forms are often generated through ion-exchange chromatography to enhance solubility in aqueous buffers .
Q. How can researchers experimentally determine the binding kinetics and affinity of this compound for target enzymes?
Advanced
Fluorescence quenching and isothermal titration calorimetry (ITC) are standard for measuring binding constants (Kd). For example, fluorescence assays monitor changes in tryptophan emission spectra upon inhibitor-enzyme interaction, while ITC quantifies enthalpy changes. NMR spectroscopy (e.g., P or H-N HSQC) provides residue-specific binding data, particularly for dynamic regions like the phosphate backbone . Surface plasmon resonance (SPR) can also assess real-time kinetics (kon/koff) using immobilized enzyme platforms .
Q. How should researchers address contradictory inhibitory data across experimental systems (e.g., varying IC50 values)?
Advanced
Contradictions often arise from differences in enzyme isoforms, buffer conditions (e.g., Mg concentration), or assay pH. To resolve these:
- Control for cofactors : Mg is critical for phosphate coordination; its absence may artificially reduce affinity .
- Structural validation : Perform X-ray crystallography or cryo-EM to confirm binding modes across isoforms (e.g., human vs. yeast adenylate kinase) .
- Activity assays : Use coupled enzymatic systems (e.g., ATPase/NADH oxidation) to rule out off-target effects .
Q. What are the key applications of this compound in studying mitochondrial energy metabolism?
Advanced
In mitochondrial studies, the compound inhibits F0F1-ATP synthase by competing with adenine nucleotide translocase (ANT). Methodological considerations include:
- Matrix conditions : Use safranine O to monitor membrane potential and Magnesium Green to quantify free Mg during inhibition assays .
- Permeabilized mitochondria : Treat with digitonin to ensure compound accessibility while preserving inner membrane integrity.
- Cross-validation : Compare results with other inhibitors (e.g., oligomycin) to confirm specificity .
Q. How can structural biology techniques elucidate the compound’s mechanism in enzyme inhibition?
Advanced
X-ray crystallography at resolutions ≤2.6 Å (e.g., PDB 1AKE) reveals atomic-level interactions, such as hydrogen bonding between the pentaphosphate and adenylate kinase’s P-loop. Molecular dynamics simulations further predict conformational changes during binding. For transient states, time-resolved crystallography or cryo-EM can capture dynamic inhibition processes .
Q. What analytical techniques validate the compound’s integrity in experimental preparations?
Basic
- HPLC : Monitor purity using a C18 column with UV detection at 260 nm (adenosine/thymidine absorbance) .
- Mass spectrometry (MS) : ESI-MS in negative ion mode confirms molecular weight (expected ~1.0 kDa) and detects hydrolysis products (e.g., triphosphate fragments) .
- P NMR : Peaks at δ -10 to -22 ppm verify intact phosphate linkages .
Q. How does this compound compare to other bisubstrate inhibitors (e.g., Ap5A) in specificity and potency?
Advanced
Unlike Ap5A (two adenosines), the adenosine-thymidine pairing in this compound confers selectivity for thymidylate kinases over adenylate kinases. Enzyme kinetics assays (e.g., competitive inhibition with varying ATP/dTMP) quantify specificity. Structural alignment tools (e.g., PyMOL) highlight steric clashes in non-target enzymes, explaining reduced off-target activity .
Comparaison Avec Des Composés Similaires
Comparison with Structurally Similar Compounds
Structural and Functional Differences
The primary structural distinction lies in the nucleoside composition:
Key Observations :
- Enzyme Specificity: The adenosine-thymidine variant demonstrates selectivity for mycobacterial thymidylate kinase (mtTMPK), while Ap5A broadly inhibits adenylate kinase across eukaryotic and prokaryotic systems .
- Inhibitory Potency: Ap5A is a well-characterized potent inhibitor of adenylate kinase (Ki in the nanomolar range), whereas the adenosine-thymidine pentaphosphate exhibits weaker inhibition in platelet adenylate kinase .
- Synthetic Challenges : Thymidine-containing polyphosphates require modified synthetic strategies due to incompatibility of standard deprotection conditions with methyl phosphate groups, as seen in thymidine triphosphate syntheses .
Biochemical and Pharmacological Data
Table 1: Comparative Biochemical Properties
Notable Findings:
- Adenylate Kinase Inhibition: Ap5A binds tightly to the active site, mimicking the transition state of ATP-AMP phosphoryl transfer .
- Role in ATP Synthase Assays : Ap5A is used to inhibit adenylate kinase in ATP synthesis assays (e.g., in Mycobacterium smegmatis), ensuring accurate measurement of ATPase activity .
Research Implications and Gaps
- Structural Insights: Molecular dynamics studies comparing Ap5A and adenosine-thymidine pentaphosphate binding could elucidate nucleoside-specific interactions in kinase inhibition.
- Data Gaps: Quantitative kinetic parameters (e.g., Ki, IC50) for the adenosine-thymidine compound remain unreported, highlighting a need for further enzymological characterization.
Propriétés
IUPAC Name |
[[(2R,3S,4R,5R)-5-(6-aminopurin-9-yl)-3,4-dihydroxyoxolan-2-yl]methoxy-hydroxyphosphoryl] [hydroxy-[hydroxy-[[(2R,3S,5R)-3-hydroxy-5-(5-methyl-2,4-dioxopyrimidin-1-yl)oxolan-2-yl]methoxy]phosphoryl]oxyphosphoryl] hydrogen phosphate | |
---|---|---|
Source | PubChem | |
URL | https://pubchem.ncbi.nlm.nih.gov | |
Description | Data deposited in or computed by PubChem | |
InChI |
InChI=1S/C20H29N7O20P4/c1-8-3-26(20(32)25-18(8)31)12-2-9(28)10(43-12)4-41-48(33,34)45-50(37,38)47-51(39,40)46-49(35,36)42-5-11-14(29)15(30)19(44-11)27-7-24-13-16(21)22-6-23-17(13)27/h3,6-7,9-12,14-15,19,28-30H,2,4-5H2,1H3,(H,33,34)(H,35,36)(H,37,38)(H,39,40)(H2,21,22,23)(H,25,31,32)/t9-,10+,11+,12+,14+,15+,19+/m0/s1 | |
Source | PubChem | |
URL | https://pubchem.ncbi.nlm.nih.gov | |
Description | Data deposited in or computed by PubChem | |
InChI Key |
WLGHSSFVEUABFP-SLFMBYJQSA-N | |
Source | PubChem | |
URL | https://pubchem.ncbi.nlm.nih.gov | |
Description | Data deposited in or computed by PubChem | |
Canonical SMILES |
CC1=CN(C(=O)NC1=O)C2CC(C(O2)COP(=O)(O)OP(=O)(O)OP(=O)(O)OP(=O)(O)OCC3C(C(C(O3)N4C=NC5=C(N=CN=C54)N)O)O)O | |
Source | PubChem | |
URL | https://pubchem.ncbi.nlm.nih.gov | |
Description | Data deposited in or computed by PubChem | |
Isomeric SMILES |
CC1=CN(C(=O)NC1=O)[C@H]2C[C@@H]([C@H](O2)COP(=O)(O)OP(=O)(O)OP(=O)(O)OP(=O)(O)OC[C@@H]3[C@H]([C@H]([C@@H](O3)N4C=NC5=C(N=CN=C54)N)O)O)O | |
Source | PubChem | |
URL | https://pubchem.ncbi.nlm.nih.gov | |
Description | Data deposited in or computed by PubChem | |
Molecular Formula |
C20H29N7O20P4 | |
Source | PubChem | |
URL | https://pubchem.ncbi.nlm.nih.gov | |
Description | Data deposited in or computed by PubChem | |
DSSTOX Substance ID |
DTXSID90158807 | |
Record name | P(1)-(Adenosine-5')-P(5)-(thymidine-5')-pentaphosphate | |
Source | EPA DSSTox | |
URL | https://comptox.epa.gov/dashboard/DTXSID90158807 | |
Description | DSSTox provides a high quality public chemistry resource for supporting improved predictive toxicology. | |
Molecular Weight |
811.4 g/mol | |
Source | PubChem | |
URL | https://pubchem.ncbi.nlm.nih.gov | |
Description | Data deposited in or computed by PubChem | |
CAS No. |
13457-68-6 | |
Record name | P(1)-(Adenosine-5')-P(5)-(thymidine-5')-pentaphosphate | |
Source | ChemIDplus | |
URL | https://pubchem.ncbi.nlm.nih.gov/substance/?source=chemidplus&sourceid=0013457686 | |
Description | ChemIDplus is a free, web search system that provides access to the structure and nomenclature authority files used for the identification of chemical substances cited in National Library of Medicine (NLM) databases, including the TOXNET system. | |
Record name | P(1)-(Adenosine-5')-P(5)-(thymidine-5')-pentaphosphate | |
Source | EPA DSSTox | |
URL | https://comptox.epa.gov/dashboard/DTXSID90158807 | |
Description | DSSTox provides a high quality public chemistry resource for supporting improved predictive toxicology. | |
Retrosynthesis Analysis
AI-Powered Synthesis Planning: Our tool employs the Template_relevance Pistachio, Template_relevance Bkms_metabolic, Template_relevance Pistachio_ringbreaker, Template_relevance Reaxys, Template_relevance Reaxys_biocatalysis model, leveraging a vast database of chemical reactions to predict feasible synthetic routes.
One-Step Synthesis Focus: Specifically designed for one-step synthesis, it provides concise and direct routes for your target compounds, streamlining the synthesis process.
Accurate Predictions: Utilizing the extensive PISTACHIO, BKMS_METABOLIC, PISTACHIO_RINGBREAKER, REAXYS, REAXYS_BIOCATALYSIS database, our tool offers high-accuracy predictions, reflecting the latest in chemical research and data.
Strategy Settings
Precursor scoring | Relevance Heuristic |
---|---|
Min. plausibility | 0.01 |
Model | Template_relevance |
Template Set | Pistachio/Bkms_metabolic/Pistachio_ringbreaker/Reaxys/Reaxys_biocatalysis |
Top-N result to add to graph | 6 |
Feasible Synthetic Routes
Avertissement et informations sur les produits de recherche in vitro
Veuillez noter que tous les articles et informations sur les produits présentés sur BenchChem sont destinés uniquement à des fins informatives. Les produits disponibles à l'achat sur BenchChem sont spécifiquement conçus pour des études in vitro, qui sont réalisées en dehors des organismes vivants. Les études in vitro, dérivées du terme latin "in verre", impliquent des expériences réalisées dans des environnements de laboratoire contrôlés à l'aide de cellules ou de tissus. Il est important de noter que ces produits ne sont pas classés comme médicaments et n'ont pas reçu l'approbation de la FDA pour la prévention, le traitement ou la guérison de toute condition médicale, affection ou maladie. Nous devons souligner que toute forme d'introduction corporelle de ces produits chez les humains ou les animaux est strictement interdite par la loi. Il est essentiel de respecter ces directives pour assurer la conformité aux normes légales et éthiques en matière de recherche et d'expérimentation.