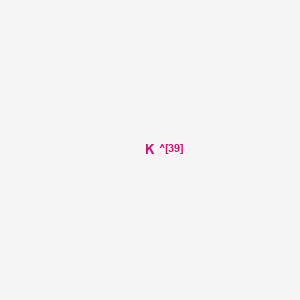
Potassium-39
Vue d'ensemble
Description
Potassium-39 (³⁹K) is the most abundant stable isotope of potassium, constituting 93.3% of natural potassium . It has 19 protons and 20 neutrons, giving it a mass number of 39 . As a spin-3/2 nucleus, ³⁹K exhibits quadrupolar interactions, which influence its magnetic resonance properties . Unlike radioactive potassium-40 (⁴⁰K), ³⁹K is non-radioactive, making it suitable for applications requiring stability, such as magnetic resonance imaging (MRI) and quantum physics experiments . Its nuclear properties, including a broad Feshbach resonance at 402 Gauss, enable precise control of atomic interactions in ultracold physics .
Méthodes De Préparation
Synthetic Routes and Reaction Conditions: Potassium-39, being a naturally occurring isotope, does not require synthetic preparation. It is typically obtained through the isolation of potassium from natural sources.
Industrial Production Methods: Potassium is primarily extracted from minerals such as sylvite (potassium chloride), carnallite (potassium magnesium chloride), and langbeinite (potassium magnesium sulfate). The extraction process involves the following steps:
Mining: Potassium-containing minerals are mined from underground deposits.
Crushing and Grinding: The mined ore is crushed and ground to liberate the potassium minerals.
Flotation: The ground ore is subjected to flotation to separate potassium minerals from other impurities.
Crystallization: The potassium-rich solution is evaporated to crystallize potassium chloride.
Purification: The crude potassium chloride is purified to obtain high-purity potassium.
Analyse Des Réactions Chimiques
Types of Reactions: Potassium-39, like other potassium isotopes, undergoes various chemical reactions, including:
Oxidation: Potassium reacts with oxygen to form potassium oxide.
Reduction: Potassium can be reduced from its compounds using strong reducing agents.
Substitution: Potassium can participate in substitution reactions, where it replaces other cations in compounds.
Common Reagents and Conditions:
Oxidation: Potassium reacts with oxygen at room temperature to form potassium oxide.
Reduction: Potassium compounds can be reduced using reducing agents such as sodium or lithium.
Substitution: Potassium can replace other cations in aqueous solutions under appropriate conditions.
Major Products:
Oxidation: Potassium oxide (K2O)
Reduction: Elemental potassium (K)
Substitution: Various potassium salts, depending on the reaction.
Applications De Recherche Scientifique
Tracing Earth Processes
Potassium isotopes, particularly , are utilized as tracers in geochemical studies. The isotopic fractionation of potassium during geological processes provides insights into:
- Chemical Weathering : Variations in concentrations help understand weathering rates and processes influencing soil formation.
- Subduction Zones : The behavior of potassium isotopes during subduction can reveal information about the recycling of materials from the Earth's surface into the mantle .
Cosmochemical Studies
In cosmochemistry, assists in studying planetary formation and solar nebula condensation. The stable isotopes of potassium are integral to understanding the evolution of planetary bodies and the conditions under which they formed .
Nutrient Cycling
The role of in biological systems is critical for understanding nutrient cycling within ecosystems. Studies have shown that plants preferentially uptake different potassium isotopes, which can be used to trace nutrient pathways and assess soil fertility .
Fertilizer Studies
This compound is also used in agricultural research to evaluate the efficiency of potassium fertilizers. By analyzing the uptake of by crops, researchers can optimize fertilizer formulations to enhance crop yields while minimizing environmental impact .
In Vivo Studies
Nuclear magnetic resonance spectroscopy techniques employing have been developed for in vivo studies, allowing scientists to investigate potassium dynamics in biological systems. This application is particularly useful in medical research for monitoring cellular functions and metabolic pathways .
Complexation Kinetics
Recent studies have utilized NMR to explore the kinetics of complexation reactions involving potassium ions with various ligands. This research helps elucidate fundamental interactions that are crucial in both organic and inorganic chemistry .
Data Table: Properties and Applications of this compound
Property | Value |
---|---|
Natural Abundance | 93.258% |
Atomic Mass | 38.963706 Da |
Neutron Number | 20 |
Proton Number | 19 |
Stability | Stable |
Applications | Geochemistry, Agriculture, NMR Spectroscopy |
Geochemical Tracing
A study published in Geochemistry examined the use of isotopes to trace weathering processes in a tropical rainforest ecosystem. The results indicated significant isotopic fractionation during chemical weathering, providing insights into nutrient release mechanisms from soils .
Agricultural Efficiency
Research conducted on rice cultivation demonstrated that using -labeled fertilizers significantly improved potassium uptake efficiency compared to traditional fertilizers, leading to a 20% increase in yield without additional environmental costs .
Mécanisme D'action
Potassium-39 exerts its effects primarily through its role as an essential intracellular cation. It is involved in maintaining cellular osmotic balance, transmitting nerve impulses, and regulating muscle contractions. Potassium ions interact with various molecular targets, including ion channels and transporters, to facilitate these physiological processes .
Comparaison Avec Des Composés Similaires
Comparison with Similar Isotopes and Compounds
Comparison with Other Potassium Isotopes
Key Findings :
- ³⁹K dominates natural potassium and is preferred for non-radioactive applications, while ⁴⁰K’s radioactivity limits its use to niche fields like geochronology .
Comparison with Other Quadrupolar Nuclei in MRI
Key Findings :
- ³⁹K MRI is less sensitive than ²³Na or ³⁵Cl MRI due to its lower gyromagnetic ratio, requiring ultrahigh-field (7 Tesla+) systems for viable imaging .
- ³⁹K’s quadrupolar interactions shorten relaxation times, complicating signal acquisition but providing unique insights into ion dynamics .
Solid-State NMR Spectroscopy
Key Findings :
- ³⁹K’s chemical shift anisotropy exceeds ²³Na’s, enabling detailed studies of cation-π interactions in biomolecules .
- Ultrahigh-field NMR (>21 Tesla) mitigates ³⁹K’s low sensitivity, revealing dynamics inaccessible via other nuclei .
Environmental and Industrial Roles
Activité Biologique
Potassium-39 () is a stable isotope of potassium that plays a critical role in various biological processes. This article explores its biological activity, focusing on its transport mechanisms, physiological significance, and implications in various biological systems.
Overview of Potassium in Biological Systems
Potassium is an essential nutrient for all living organisms, serving as a key cation involved in numerous cellular functions, including:
- Cellular homeostasis : Maintaining osmotic balance and membrane potential.
- Signal transduction : Participating in nerve impulse transmission and muscle contraction.
- Enzyme activation : Acting as a cofactor for various enzymatic reactions.
The natural abundance of potassium isotopes includes (93.258%) and (6.730%), with a typical natural ratio of approximately 0.07217 for .
Transport Mechanisms of this compound
Research indicates that the fractionation of potassium isotopes, particularly , during transport processes is influenced by the specific pathways and transport machinery involved.
Key Findings on Transport Mechanisms
- Transcellular Transport :
- Paracellular Transport :
- Nuclear Magnetic Resonance (NMR) Studies :
Case Study 1: Rat Model Analysis
A study involving Rattus norvegicus (common rat) examined the isotopic composition of potassium in various tissues. The findings included:
- A positive enrichment of was observed in feces compared to urine, indicating preferential absorption across the gut epithelium.
- Tissue analysis showed that muscle tissues had varying δ-values for relative to blood plasma, suggesting differential uptake mechanisms influenced by Na+/K+ ATPase activity .
Tissue Type | δ-value (‰) | Significance |
---|---|---|
Gastrocnemius Muscle | +0.01 ± 0.15 | Neutral uptake relative to plasma |
Extensor Digitorum Longus | +0.17 ± 0.11 | Slight enrichment indicating active transport |
Urine | -0.50 ± 0.10 | Significant enrichment of |
Case Study 2: Algal Systems
In studies involving Chlamydomonas reinhardtii (a green alga), it was found that:
- The δ-value for whole cells was significantly lower than that of the growth medium, indicating active uptake mechanisms favoring .
- This research highlights the role of potassium isotopes in understanding nutrient dynamics within aquatic ecosystems .
Implications for Health and Disease
The biological activity of potassium isotopes extends beyond basic cellular functions; it has implications for health and disease management:
- Cardiovascular Health : Adequate potassium levels are crucial for heart function, as evidenced by studies showing that metabolic inhibition affects intracellular potassium levels significantly .
- Neurotransmission : Potassium's role in nerve impulse transmission underscores its importance in neurological health.
Q & A
Q. Basic: What methods are used to determine the isotopic composition of potassium-39 in natural samples?
Answer:
The isotopic abundance of this compound (K-39) is calculated using mass spectrometry and atomic mass equations. For example, the relative abundance of K-39 (~93.2%) and K-41 (~6.8%) is derived by solving the equation:
where is the fractional abundance of K-38. This method requires precise calibration of mass spectrometers and validation against certified reference materials to minimize systematic errors .
Q. Basic: What spectroscopic techniques are employed to study this compound in aqueous solutions?
Answer:
Nuclear magnetic resonance (NMR) spectroscopy, particularly quadrupolar relaxation studies of , is used to probe ion dynamics in solutions. For example, NMR can reveal subpicosecond collective motion in electrolytes by analyzing relaxation rates, which depend on ion hydration and electric field gradients. Experimental protocols require high-field NMR spectrometers and temperature-controlled probes to ensure reproducibility .
Q. Advanced: How do researchers resolve contradictions in isotopic abundance data for this compound across analytical methods?
Answer:
Discrepancies between mass spectrometry, neutron activation analysis, and optical emission spectroscopy are addressed through interlaboratory comparisons and error source analysis. For instance, neutron flux variations in activation analysis can skew K-39 measurements, requiring normalization to standard samples like NIST SRM 999b. Statistical tools like weighted mean calculations and uncertainty propagation models are applied to reconcile data .
Q. Advanced: What experimental design considerations are critical for potassium-argon dating using this compound?
Answer:
In K-Ar dating, neutron irradiation converts K-39 to argon-39 (), which is quantified via mass spectrometry. Key considerations include:
- Sample purity : Removal of atmospheric argon contaminants.
- Neutron flux calibration : Use of flux monitors (e.g., ) to account for spatial variations in irradiation.
- Isotopic correction : Adjustments for from radioactive decay and from atmospheric sources.
Methodological rigor ensures age estimates with uncertainties <1% .
Q. Advanced: How does this compound NMR relaxation inform ion dynamics in biological systems?
Answer:
Quadrupolar NMR measures relaxation rates sensitive to ion-protein interactions and hydration dynamics. For example, in extracellular fluid studies, relaxation dispersion experiments reveal ion binding kinetics to macromolecules. Advanced pulse sequences (e.g., Carr-Purcell-Meiboom-Gill) mitigate signal decay artifacts, while molecular dynamics simulations validate experimental data .
Q. Advanced: What challenges arise in modeling this compound’s nuclear properties for astrophysical studies?
Answer:
Theoretical models of K-39 nucleosynthesis (e.g., in supernova neutron capture processes) often conflict with experimental cross-section data. For example, the reaction rate discrepancies are addressed using tandem accelerator experiments and R-matrix theory. Iterative refinement of nuclear potential parameters (e.g., Woods-Saxon depths) improves agreement between predicted and observed isotopic abundances .
Q. Advanced: How do isotopic fractionation effects impact this compound quantification in environmental samples?
Answer:
Natural fractionation of K-39 during biogeochemical processes (e.g., plant uptake, mineral weathering) is measured via high-resolution multi-collector ICP-MS. Researchers apply isotope dilution with -enriched spikes to correct for matrix effects. Kinetic fractionation models, such as Rayleigh distillation equations, are used to interpret soil-plant-atmosphere cycling data .
Propriétés
IUPAC Name |
potassium-39 | |
---|---|---|
Source | PubChem | |
URL | https://pubchem.ncbi.nlm.nih.gov | |
Description | Data deposited in or computed by PubChem | |
InChI |
InChI=1S/K/i1+0 | |
Source | PubChem | |
URL | https://pubchem.ncbi.nlm.nih.gov | |
Description | Data deposited in or computed by PubChem | |
InChI Key |
ZLMJMSJWJFRBEC-IGMARMGPSA-N | |
Source | PubChem | |
URL | https://pubchem.ncbi.nlm.nih.gov | |
Description | Data deposited in or computed by PubChem | |
Canonical SMILES |
[K] | |
Source | PubChem | |
URL | https://pubchem.ncbi.nlm.nih.gov | |
Description | Data deposited in or computed by PubChem | |
Isomeric SMILES |
[39K] | |
Source | PubChem | |
URL | https://pubchem.ncbi.nlm.nih.gov | |
Description | Data deposited in or computed by PubChem | |
Molecular Formula |
K | |
Source | PubChem | |
URL | https://pubchem.ncbi.nlm.nih.gov | |
Description | Data deposited in or computed by PubChem | |
DSSTOX Substance ID |
DTXSID30930947 | |
Record name | (~39~K)Potassium | |
Source | EPA DSSTox | |
URL | https://comptox.epa.gov/dashboard/DTXSID30930947 | |
Description | DSSTox provides a high quality public chemistry resource for supporting improved predictive toxicology. | |
Molecular Weight |
38.96370648 g/mol | |
Source | PubChem | |
URL | https://pubchem.ncbi.nlm.nih.gov | |
Description | Data deposited in or computed by PubChem | |
CAS No. |
14092-91-2 | |
Record name | (~39~K)Potassium | |
Source | EPA DSSTox | |
URL | https://comptox.epa.gov/dashboard/DTXSID30930947 | |
Description | DSSTox provides a high quality public chemistry resource for supporting improved predictive toxicology. | |
Synthesis routes and methods I
Procedure details
Synthesis routes and methods II
Procedure details
Synthesis routes and methods III
Procedure details
Avertissement et informations sur les produits de recherche in vitro
Veuillez noter que tous les articles et informations sur les produits présentés sur BenchChem sont destinés uniquement à des fins informatives. Les produits disponibles à l'achat sur BenchChem sont spécifiquement conçus pour des études in vitro, qui sont réalisées en dehors des organismes vivants. Les études in vitro, dérivées du terme latin "in verre", impliquent des expériences réalisées dans des environnements de laboratoire contrôlés à l'aide de cellules ou de tissus. Il est important de noter que ces produits ne sont pas classés comme médicaments et n'ont pas reçu l'approbation de la FDA pour la prévention, le traitement ou la guérison de toute condition médicale, affection ou maladie. Nous devons souligner que toute forme d'introduction corporelle de ces produits chez les humains ou les animaux est strictement interdite par la loi. Il est essentiel de respecter ces directives pour assurer la conformité aux normes légales et éthiques en matière de recherche et d'expérimentation.