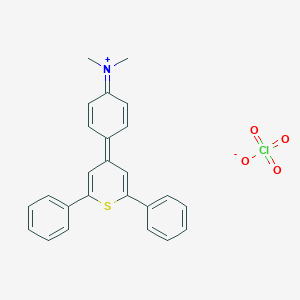
Ionophore d'iodure I
- Cliquez sur DEMANDE RAPIDE pour recevoir un devis de notre équipe d'experts.
- Avec des produits de qualité à un prix COMPÉTITIF, vous pouvez vous concentrer davantage sur votre recherche.
Vue d'ensemble
Description
Iodide ionophore I is a useful research compound. Its molecular formula is C25H22ClNO4S and its molecular weight is 468 g/mol. The purity is usually 95%.
The exact mass of the compound Iodide ionophore I is unknown and the complexity rating of the compound is unknown. The United Nations designated GHS hazard class pictogram is Irritant, and the GHS signal word is WarningThe storage condition is unknown. Please store according to label instructions upon receipt of goods.
BenchChem offers high-quality Iodide ionophore I suitable for many research applications. Different packaging options are available to accommodate customers' requirements. Please inquire for more information about Iodide ionophore I including the price, delivery time, and more detailed information at info@benchchem.com.
Applications De Recherche Scientifique
Surveillance environnementale
Les ISE, y compris ceux utilisant l'ionophore d'iodure I, sont utilisés pour déterminer les ions dans les échantillons d'eau environnementaux . Ils aident à surveiller la qualité de l'eau en détectant la présence d'ions spécifiques .
Applications cliniques
Dans le domaine médical, les ISE sont utilisés pour la détection des ions dans les fluides biologiques . Ceci est crucial dans le diagnostic et la surveillance de diverses conditions de santé .
Contrôle de la qualité alimentaire
Les ISE sont également utilisés dans l'industrie alimentaire pour la détermination des ions dans les échantillons alimentaires . Cela contribue à garantir la sécurité et la qualité des produits alimentaires .
Analyses de l'iode urinaire
Les capteurs d'iodure potentiométriques à base d'this compound sont très applicables aux analyses de l'iode urinaire . L'iode urinaire est un marqueur biochimique du contrôle des troubles liés à une carence en iode .
Biotechnologie
En biotechnologie, les ionophores sont utilisés pour moduler ou améliorer le rôle des ions clés dans la cellule . Ceci est crucial dans diverses applications biotechnologiques
Mécanisme D'action
Target of Action
Iodide ionophores, like Iodide Ionophore I, primarily target biological membranes . These ionophores are complex natural products known to transport various cations across biological membranes . They are typically grouped as having similar functions, despite the fact that they significantly differ in structure .
Analyse Biochimique
Biochemical Properties
Iodide Ionophore I interacts with several biomolecules, including enzymes and proteins, to facilitate the transport of iodide ions. It is highly selective and sensitive to iodide, making it an essential tool in biochemical assays . The compound’s interaction with these biomolecules is primarily through the formation of complexes, which are then transported across biological membranes .
Cellular Effects
Iodide Ionophore I has a profound impact on various types of cells and cellular processes. It influences cell function by altering the concentration gradient for ions in microorganisms, which can disrupt the membrane potential and exhibit cytotoxic properties . It also plays a role in the regulation of cell signaling pathways, gene expression, and cellular metabolism .
Molecular Mechanism
The molecular mechanism of Iodide Ionophore I involves the formation of complexes with ions, which are then transported across biological membranes . This process is facilitated by the compound’s lipophilic nature, which prevents it from being leached from the membrane upon exposure to aqueous solutions . The compound can also affect gene expression and enzyme activity, contributing to its overall effects at the molecular level .
Temporal Effects in Laboratory Settings
In laboratory settings, the effects of Iodide Ionophore I can change over time. The compound exhibits high selectivity and sensitivity to iodide, making it suitable for assays involving large populations . Information on the product’s stability, degradation, and long-term effects on cellular function can be observed in in vitro or in vivo studies .
Dosage Effects in Animal Models
The effects of Iodide Ionophore I can vary with different dosages in animal models. For instance, ionophores have been used in cattle diets to alter ruminal fermentation dynamics and improve feed efficiency . Species-specific variability exists in the median lethal dose for ionophores, indicating potential toxic or adverse effects at high doses .
Metabolic Pathways
Iodide Ionophore I is involved in several metabolic pathways. It interacts with enzymes and cofactors, affecting metabolic flux or metabolite levels . The compound’s primary role is as a constituent of thyroid hormones, which control basal metabolism, growth, and development .
Transport and Distribution
Iodide Ionophore I is transported and distributed within cells and tissues through various transporters. It is principally transported by the sodium iodide symporter (NIS) and by the anion exchanger PENDRIN . The compound’s effects on its localization or accumulation are also significant .
Subcellular Localization
The subcellular localization of Iodide Ionophore I and its effects on activity or function are crucial. The compound is predominantly found at the plasma membrane in some organisms, while it is intracellular in others . This localization can influence the compound’s activity and function .
Activité Biologique
Iodide ionophores, particularly Iodide Ionophore I (XB1) , have garnered attention for their unique ability to selectively transport iodide ions across biological membranes. This property is critical due to the essential role of iodide in various physiological processes, including thyroid hormone synthesis, metabolism regulation, and neurological function. This article explores the biological activity of Iodide Ionophore I, focusing on its mechanisms of action, applications in sensing technologies, and potential therapeutic uses.
Iodide Ionophore I operates primarily through halogen bonding interactions , which facilitate the selective binding and transport of iodide ions. NMR studies have demonstrated that XB1 exhibits a high binding affinity for iodide, with a dissociation constant K11=(260±10)M−1, indicating a strong interaction between the ionophore and the iodide ion . The mechanism involves the formation of a stable complex between XB1 and I− ions, which enhances the efficiency of iodide transport across lipid membranes.
Biological Significance
Iodide is an essential micronutrient required for the synthesis of thyroid hormones. Deficiencies in iodide can lead to various health issues, including goiter and hypothyroidism. The ability of Iodide Ionophore I to facilitate rapid iodide transport makes it a valuable tool in both clinical and research settings for assessing iodide levels in biological samples .
Applications in Sensing Technologies
Recent studies have highlighted the application of XB1 in developing potentiometric sensors for iodide detection. These sensors leverage the ionophore's selectivity to provide accurate measurements of iodide concentrations in complex matrices such as food and biological fluids. The optimal electrode configuration using XB1 has shown a near Nernstian response of −51.9 mV per decade over a dynamic range from 10−1 to 10−6M . This capability is significant for monitoring dietary iodide intake and assessing thyroid health.
Case Study 1: Potentiometric Sensing
A study by Seah et al. demonstrated the effectiveness of XB1 in creating selective electrodes for iodide sensing. The research indicated that XB1 provided superior selectivity for iodide over competing anions such as thiocyanate, with selectivity coefficients indicating a strong preference for I− ions . This study underscores the potential of iodide ionophores in clinical diagnostics.
Comparative Analysis
Property | Iodide Ionophore I (XB1) | Other Ionophores |
---|---|---|
Selectivity | High for I− | Varies widely |
Binding Affinity | K11=260M−1 | Typically lower |
Applications | Potentiometric sensing | Antimicrobial, therapeutic |
Mechanism | Halogen bonding | Varies (e.g., cation transport) |
Propriétés
IUPAC Name |
[4-(2,6-diphenylthiopyran-4-ylidene)cyclohexa-2,5-dien-1-ylidene]-dimethylazanium;perchlorate |
Source
|
---|---|---|
Source | PubChem | |
URL | https://pubchem.ncbi.nlm.nih.gov | |
Description | Data deposited in or computed by PubChem | |
InChI |
InChI=1S/C25H22NS.ClHO4/c1-26(2)23-15-13-19(14-16-23)22-17-24(20-9-5-3-6-10-20)27-25(18-22)21-11-7-4-8-12-21;2-1(3,4)5/h3-18H,1-2H3;(H,2,3,4,5)/q+1;/p-1 |
Source
|
Source | PubChem | |
URL | https://pubchem.ncbi.nlm.nih.gov | |
Description | Data deposited in or computed by PubChem | |
InChI Key |
WPXOZDYAIYMJBU-UHFFFAOYSA-M |
Source
|
Source | PubChem | |
URL | https://pubchem.ncbi.nlm.nih.gov | |
Description | Data deposited in or computed by PubChem | |
Canonical SMILES |
C[N+](=C1C=CC(=C2C=C(SC(=C2)C3=CC=CC=C3)C4=CC=CC=C4)C=C1)C.[O-]Cl(=O)(=O)=O |
Source
|
Source | PubChem | |
URL | https://pubchem.ncbi.nlm.nih.gov | |
Description | Data deposited in or computed by PubChem | |
Molecular Formula |
C25H22ClNO4S |
Source
|
Source | PubChem | |
URL | https://pubchem.ncbi.nlm.nih.gov | |
Description | Data deposited in or computed by PubChem | |
Molecular Weight |
468.0 g/mol |
Source
|
Source | PubChem | |
URL | https://pubchem.ncbi.nlm.nih.gov | |
Description | Data deposited in or computed by PubChem | |
CAS No. |
14039-00-0 |
Source
|
Record name | Iodide ionophore I | |
Source | European Chemicals Agency (ECHA) | |
URL | https://echa.europa.eu/information-on-chemicals | |
Description | The European Chemicals Agency (ECHA) is an agency of the European Union which is the driving force among regulatory authorities in implementing the EU's groundbreaking chemicals legislation for the benefit of human health and the environment as well as for innovation and competitiveness. | |
Explanation | Use of the information, documents and data from the ECHA website is subject to the terms and conditions of this Legal Notice, and subject to other binding limitations provided for under applicable law, the information, documents and data made available on the ECHA website may be reproduced, distributed and/or used, totally or in part, for non-commercial purposes provided that ECHA is acknowledged as the source: "Source: European Chemicals Agency, http://echa.europa.eu/". Such acknowledgement must be included in each copy of the material. ECHA permits and encourages organisations and individuals to create links to the ECHA website under the following cumulative conditions: Links can only be made to webpages that provide a link to the Legal Notice page. | |
Avertissement et informations sur les produits de recherche in vitro
Veuillez noter que tous les articles et informations sur les produits présentés sur BenchChem sont destinés uniquement à des fins informatives. Les produits disponibles à l'achat sur BenchChem sont spécifiquement conçus pour des études in vitro, qui sont réalisées en dehors des organismes vivants. Les études in vitro, dérivées du terme latin "in verre", impliquent des expériences réalisées dans des environnements de laboratoire contrôlés à l'aide de cellules ou de tissus. Il est important de noter que ces produits ne sont pas classés comme médicaments et n'ont pas reçu l'approbation de la FDA pour la prévention, le traitement ou la guérison de toute condition médicale, affection ou maladie. Nous devons souligner que toute forme d'introduction corporelle de ces produits chez les humains ou les animaux est strictement interdite par la loi. Il est essentiel de respecter ces directives pour assurer la conformité aux normes légales et éthiques en matière de recherche et d'expérimentation.