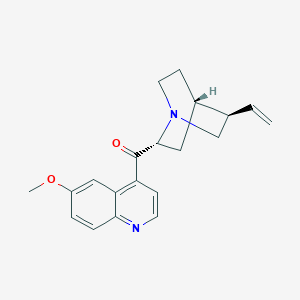
Quinidinone
Vue d'ensemble
Description
Quinidinone is a natural product found in Cinchona . It has the molecular formula C20H22N2O2 and a molecular weight of 322.4 g/mol .
Synthesis Analysis
In addition to the well-known quinidine metabolites, 2’-quinidinone and 3-hydroxy-quinidine, a third metabolic product was detected in the plasma of cardiac patients receiving quinidine therapy . The structure of quinidine-N-oxide for the hitherto unknown metabolite was finally confirmed by X-ray diffraction analysis .
Molecular Structure Analysis
Quinidinone has a complex molecular structure. Its IUPAC name is [(2R,4S,5R)-5-ethenyl-1-azabicyclo[2.2.2]octan-2-yl]-(6-methoxyquinolin-4-yl)methanone . The InChI and SMILES strings provide more detailed information about its structure .
Chemical Reactions Analysis
Quinones are fast redox cycling molecules and have the potential to bind to thiol, amine, and hydroxyl groups . They are known to be chemically reactive .
Physical And Chemical Properties Analysis
Quinidinone has a molecular weight of 322.4 g/mol . More detailed physical and chemical properties may require specific experimental measurements.
Applications De Recherche Scientifique
Chemical Synthesis and Structural Analysis : Quinidinone has been a subject of interest in structural biochemistry. Pettit and Gupta (1968) explored the lithium aluminum hydride reduction of oximes derived from quinidinone to synthesize 9-amino-(9-deoxy)quinidine, proposing tentative stereochemical assignments based on optical rotatory dispersion measurements (Pettit & Gupta, 1968).
Metabolism in Drug Therapy : Quinidinone is also significant as a metabolite of quinidine, a medication used for cardiac conditions. Guentert, Daly, and Riegelman (2010) identified a new metabolite of quinidine, alongside known metabolites like 2'-quinidinone, suggesting the presence of an aliphatic N-oxide group in this new compound (Guentert et al., 2010).
Analytical Methods for Quantification : Bonora, Guentert, Upton, and Riegelman (1979) developed a reverse-phase high-pressure liquid chromatography assay for quantifying urinary levels of quinidine and its major metabolites, including 2'-quinidinone (Bonora et al., 1979).
Cardiac Treatment Applications : Earlier studies, like those by Smith and Clarke (1925) and Viko, Marvin, and White (1923), discussed the use of quinidin (and by extension, its derivatives like quinidinone) in treating auricular fibrillation, highlighting its efficacy and safety in clinical settings (Smith & Clarke, 1925), (Viko et al., 1923).
Pharmacokinetic and Pharmacodynamic Studies : Studies by Guentert, Coates, Upton, Combs, and Riegelman (1979) and Polak (2013) have focused on the pharmacokinetics and pharmacodynamics of quinidine and its metabolites, including quinidinone, to understand their behavior in the human body (Guentert et al., 1979), (Polak, 2013).
Toxicological Studies and Safety Profiles : Research has also been conducted to understand the toxicological profile of quinidine and its derivatives. Levy (1922) and Selzer and Wray (1964) investigated the clinical toxicology of quinidin, providing insights into its safety and potential adverse effects (Levy, 1922), (Selzer & Wray, 1964).
Mécanisme D'action
Safety and Hazards
Propriétés
IUPAC Name |
[(2R,4S,5R)-5-ethenyl-1-azabicyclo[2.2.2]octan-2-yl]-(6-methoxyquinolin-4-yl)methanone | |
---|---|---|
Source | PubChem | |
URL | https://pubchem.ncbi.nlm.nih.gov | |
Description | Data deposited in or computed by PubChem | |
InChI |
InChI=1S/C20H22N2O2/c1-3-13-12-22-9-7-14(13)10-19(22)20(23)16-6-8-21-18-5-4-15(24-2)11-17(16)18/h3-6,8,11,13-14,19H,1,7,9-10,12H2,2H3/t13-,14-,19+/m0/s1 | |
Source | PubChem | |
URL | https://pubchem.ncbi.nlm.nih.gov | |
Description | Data deposited in or computed by PubChem | |
InChI Key |
SRFCUPVBYYAMIL-CKFHNAJUSA-N | |
Source | PubChem | |
URL | https://pubchem.ncbi.nlm.nih.gov | |
Description | Data deposited in or computed by PubChem | |
Canonical SMILES |
COC1=CC2=C(C=CN=C2C=C1)C(=O)C3CC4CCN3CC4C=C | |
Source | PubChem | |
URL | https://pubchem.ncbi.nlm.nih.gov | |
Description | Data deposited in or computed by PubChem | |
Isomeric SMILES |
COC1=CC2=C(C=CN=C2C=C1)C(=O)[C@H]3C[C@@H]4CCN3C[C@@H]4C=C | |
Source | PubChem | |
URL | https://pubchem.ncbi.nlm.nih.gov | |
Description | Data deposited in or computed by PubChem | |
Molecular Formula |
C20H22N2O2 | |
Source | PubChem | |
URL | https://pubchem.ncbi.nlm.nih.gov | |
Description | Data deposited in or computed by PubChem | |
DSSTOX Substance ID |
DTXSID301021294 | |
Record name | Quinidinone | |
Source | EPA DSSTox | |
URL | https://comptox.epa.gov/dashboard/DTXSID301021294 | |
Description | DSSTox provides a high quality public chemistry resource for supporting improved predictive toxicology. | |
Molecular Weight |
322.4 g/mol | |
Source | PubChem | |
URL | https://pubchem.ncbi.nlm.nih.gov | |
Description | Data deposited in or computed by PubChem | |
Product Name |
Quinidinone | |
CAS RN |
14528-53-1 | |
Record name | 6′-Methoxycinchonan-9-one | |
Source | CAS Common Chemistry | |
URL | https://commonchemistry.cas.org/detail?cas_rn=14528-53-1 | |
Description | CAS Common Chemistry is an open community resource for accessing chemical information. Nearly 500,000 chemical substances from CAS REGISTRY cover areas of community interest, including common and frequently regulated chemicals, and those relevant to high school and undergraduate chemistry classes. This chemical information, curated by our expert scientists, is provided in alignment with our mission as a division of the American Chemical Society. | |
Explanation | The data from CAS Common Chemistry is provided under a CC-BY-NC 4.0 license, unless otherwise stated. | |
Record name | Quinidinone | |
Source | ChemIDplus | |
URL | https://pubchem.ncbi.nlm.nih.gov/substance/?source=chemidplus&sourceid=0014528531 | |
Description | ChemIDplus is a free, web search system that provides access to the structure and nomenclature authority files used for the identification of chemical substances cited in National Library of Medicine (NLM) databases, including the TOXNET system. | |
Record name | Quinidinone | |
Source | EPA DSSTox | |
URL | https://comptox.epa.gov/dashboard/DTXSID301021294 | |
Description | DSSTox provides a high quality public chemistry resource for supporting improved predictive toxicology. | |
Record name | 6'-methoxycinchonan-9-one | |
Source | European Chemicals Agency (ECHA) | |
URL | https://echa.europa.eu/substance-information/-/substanceinfo/100.035.029 | |
Description | The European Chemicals Agency (ECHA) is an agency of the European Union which is the driving force among regulatory authorities in implementing the EU's groundbreaking chemicals legislation for the benefit of human health and the environment as well as for innovation and competitiveness. | |
Explanation | Use of the information, documents and data from the ECHA website is subject to the terms and conditions of this Legal Notice, and subject to other binding limitations provided for under applicable law, the information, documents and data made available on the ECHA website may be reproduced, distributed and/or used, totally or in part, for non-commercial purposes provided that ECHA is acknowledged as the source: "Source: European Chemicals Agency, http://echa.europa.eu/". Such acknowledgement must be included in each copy of the material. ECHA permits and encourages organisations and individuals to create links to the ECHA website under the following cumulative conditions: Links can only be made to webpages that provide a link to the Legal Notice page. | |
Synthesis routes and methods
Procedure details
Retrosynthesis Analysis
AI-Powered Synthesis Planning: Our tool employs the Template_relevance Pistachio, Template_relevance Bkms_metabolic, Template_relevance Pistachio_ringbreaker, Template_relevance Reaxys, Template_relevance Reaxys_biocatalysis model, leveraging a vast database of chemical reactions to predict feasible synthetic routes.
One-Step Synthesis Focus: Specifically designed for one-step synthesis, it provides concise and direct routes for your target compounds, streamlining the synthesis process.
Accurate Predictions: Utilizing the extensive PISTACHIO, BKMS_METABOLIC, PISTACHIO_RINGBREAKER, REAXYS, REAXYS_BIOCATALYSIS database, our tool offers high-accuracy predictions, reflecting the latest in chemical research and data.
Strategy Settings
Precursor scoring | Relevance Heuristic |
---|---|
Min. plausibility | 0.01 |
Model | Template_relevance |
Template Set | Pistachio/Bkms_metabolic/Pistachio_ringbreaker/Reaxys/Reaxys_biocatalysis |
Top-N result to add to graph | 6 |
Feasible Synthetic Routes
Q & A
Q1: What is the structural characterization of Quinidinone?
A1: While the provided research papers don't explicitly state the molecular formula and weight of Quinidinone, they focus on its relationship to Cinchona alkaloids like Quinine and Quinidine. Quinidinone is a derivative of these alkaloids, formed through specific chemical transformations. These papers delve into the structural analysis of Quinidinone, primarily using Nuclear Magnetic Resonance (NMR) spectroscopy. [, ] This technique helps determine the structure and study the tautomeric behavior of Quinidinone. []
Q2: How is Quinidinone synthesized?
A2: Several synthesis routes for Quinidinone are presented in the research papers:
- Oxidation of Quinidine: Quinidinone can be synthesized through the electrochemical oxidation of Quinidine. []
- Reaction with Metal Ketyl: A process involving the reaction of Cinchona alkaloids (including Quinine, epiQuinine, Quinidine, epiQuinidine, or their mixtures) with a metal ketyl in an inert hydrocarbon solvent yields Quinidinone (as a mixture with Quininone). []
- From d-Quinotoxine: While not directly synthesizing Quinidinone, the classic Rabe-Kindler conversion of d-Quinotoxine to Quinine involves "quininone" as an intermediate. This "quininone" is likely a mixture of Quininone and Quinidinone. []
Q3: What is the reactivity of Quinidinone in superacidic environments?
A3: Research indicates that Quinidinone undergoes both rearrangement and fluorination reactions when exposed to superacidic conditions. [, ] This unique reactivity is attributed to the specific chemical properties of superacids, leading to the formation of novel fluorinated and rearranged compounds with potentially valuable properties. [, , ]
Q4: Can Quinidinone be metabolized?
A4: One study identifies 2'-Quinidinone as a metabolite of Quinidine in rabbits. [] This finding suggests that the 2'-oxo position of Quinidine is susceptible to metabolic transformations. The study also observes other metabolites of Quinidine, indicating a more complex metabolic pathway.
Q5: Does Quinidinone exhibit cytotoxic activity?
A5: In vitro cytotoxicity testing using guinea-pig ear keratinocytes revealed that Quinidinone exhibits some level of cytotoxicity. [] This suggests potential for further investigation into its effects on cell viability and potential applications.
Q6: What is the role of Quinidinone in understanding drug-dependent antibody reactions?
A6: Research on drug-induced immunologic thrombocytopenia (DITP) explored the reactivity of Quinidine-dependent antibodies with Quinidinone. [] The study found that these antibodies showed significantly weaker reactions with Quinidinone compared to the parent drug, Quinidine. This suggests that while Quinidinone might interact with these antibodies, the native Quinidine structure is crucial for their full activity. []
Avertissement et informations sur les produits de recherche in vitro
Veuillez noter que tous les articles et informations sur les produits présentés sur BenchChem sont destinés uniquement à des fins informatives. Les produits disponibles à l'achat sur BenchChem sont spécifiquement conçus pour des études in vitro, qui sont réalisées en dehors des organismes vivants. Les études in vitro, dérivées du terme latin "in verre", impliquent des expériences réalisées dans des environnements de laboratoire contrôlés à l'aide de cellules ou de tissus. Il est important de noter que ces produits ne sont pas classés comme médicaments et n'ont pas reçu l'approbation de la FDA pour la prévention, le traitement ou la guérison de toute condition médicale, affection ou maladie. Nous devons souligner que toute forme d'introduction corporelle de ces produits chez les humains ou les animaux est strictement interdite par la loi. Il est essentiel de respecter ces directives pour assurer la conformité aux normes légales et éthiques en matière de recherche et d'expérimentation.