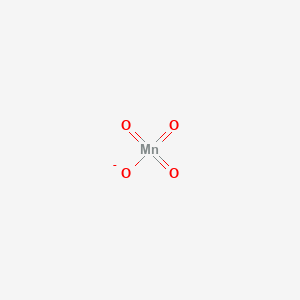
Permanganate
- Cliquez sur DEMANDE RAPIDE pour recevoir un devis de notre équipe d'experts.
- Avec des produits de qualité à un prix COMPÉTITIF, vous pouvez vous concentrer davantage sur votre recherche.
Vue d'ensemble
Description
Permanganate (MnO₄⁻) is a strongly oxidizing anion widely used in environmental remediation, water treatment, and organic synthesis. It is typically administered as potassium this compound (KMnO₄) or sodium this compound (NaMnO₄), both of which dissociate in water to release the this compound ion. Industrially, KMnO₄ is synthesized via a two-stage process: (1) oxidation of manganese dioxide (MnO₂) to potassium manganate (K₂MnO₄) in concentrated KOH, and (2) electrochemical oxidation of K₂MnO₄ to KMnO₄ .
This compound oxidizes organic contaminants via electron transfer, targeting functional groups such as alkenes, phenols, and anilines. Its effectiveness persists across a wide pH range (3.5–12), making it versatile for groundwater and industrial applications .
Q & A
Basic Research Questions
Q. How can permanganate titration methods be optimized to minimize systematic errors in redox analysis?
Redox titrations using KMnO₄ require strict control of acidity, temperature, and indicator selection. For example, in Fe²⁺ quantification, deviations in this compound molarity or analyte volume can skew results. A methodological approach involves validating titrant concentration via standardization with oxalic acid and using blank corrections to account for background oxidant demand . Error analysis should quantify uncertainties from pipetting, endpoint detection, and side reactions (e.g., MnO₂ precipitation in neutral conditions).
Q. What factors determine the reaction rate constants of this compound with organic contaminants?
Reactivity depends on the electronic structure of the target molecule. Alkenes and electron-rich groups (e.g., phenols, anilines) exhibit higher rate constants due to electrophilic attack by MnO₄⁻. Electron-withdrawing groups (e.g., nitro) suppress reactivity. Solution conditions (pH, ionic strength, buffer composition) also modulate rates. For instance, phosphate buffers may act as ligands, altering this compound’s redox potential .
Q. How can spectrophotometric methods improve accuracy in this compound-based quantitative analysis?
UV-Vis spectroscopy exploits this compound’s absorption maxima at 526–546 nm (ε ≈ 2200–2280 L·mol⁻¹·cm⁻¹). Calibration curves should account for matrix effects (e.g., dissolved organics) and interferences from reduced manganese species (e.g., MnO₂). Hydrazine determination via this compound reduction exemplifies rigorous validation by comparing absorbance changes against standardized reaction conditions .
Q. What are the limitations of using this compound for qualitative alkene detection in complex matrices?
Baeyer’s test (decolorization of KMnO₄ in basic conditions) is effective for isolated alkenes but less reliable in heterogeneous systems. Competing reductants (e.g., sulfides) or steric hindrance in substituted alkenes can yield false negatives. Confirmatory techniques like GC-MS or FTIR are recommended for ambiguous cases .
Advanced Research Questions
Q. How can Box-Behnken experimental design optimize this compound-driven oxidation processes?
Response surface methodology (RSM) with variables like temperature, catalyst loading, and reactant ratios identifies optimal conditions. For example, cyclohexanone oxime hydrolysis achieved >95% efficiency by modeling interactions between temperature (X₁), catalyst dose (X₂), and water content (X₃). Quadratic models predict maxima/minima, while ANOVA validates factor significance .
Q. What experimental strategies address contradictions in buffer-induced this compound reactivity?
Conflicting reports on buffer effects (e.g., phosphate vs. carbonate) require controlled studies isolating ionic strength vs. ligand interactions. Dual experiments—varying buffer concentration while maintaining ionic strength with inert salts (e.g., KCl)—can decouple these factors. Kinetic modeling further distinguishes diffusion-limited vs. chemically controlled regimes .
Q. How does natural oxidant demand (NOD) influence this compound dosing in groundwater remediation?
NOD arises from reactions with aquifer minerals (e.g., Fe²⁺, sulfides) and organic carbon. Batch and column studies reveal biphasic consumption: rapid initial demand (7 g KMnO₄/kg/day) followed by slow, persistent oxidation (0.005 g/kg/day). Field-scale designs must account for delayed MnO₄⁻ breakthrough and overestimation errors from total organic carbon assays .
Q. What mechanisms enhance this compound activation in catalytic membrane systems?
Carbon nanotube (CNT) membranes increase interfacial electron transfer, promoting MnO₄⁻ → Mn(III)/Mn(IV) transitions. Operando spectroscopy and DFT calculations show CNT defects act as active sites, lowering activation energy for micropollutant degradation. Optimization involves tuning pore size, flow rate, and MnO₄⁻ concentration to balance residence time and oxidant utilization .
Q. How do data discrepancies in this compound oxidation studies arise, and how can they be resolved?
Inconsistent rate constants often stem from unreported experimental details (e.g., buffer type, purity of organics). Meta-analyses of 215 compounds highlight the need for standardized protocols (fixed pH, ionic strength). Discrepancies in actinide removal efficiency (e.g., Am vs. Pu decontamination) further underscore matrix-specific validation .
Q. What analytical frameworks elucidate oxidation pathways and intermediate toxicity in this compound systems?
LC-HRMS and QSAR models identify transformation products (e.g., ketones from alkenes, nitro derivatives from anilines). Toxicity assays (e.g., Vibrio fischeri bioassays) reveal intermediates like quinones may exhibit higher ecotoxicity than parent compounds. Pathway elucidation requires coupling kinetic data with frontier molecular orbital theory to predict reactive sites .
Comparaison Avec Des Composés Similaires
Potassium Permanganate vs. Sodium this compound
Property | Potassium this compound (KMnO₄) | Sodium this compound (NaMnO₄) |
---|---|---|
Physical Form | Purple crystalline solid | Liquid solution |
Solubility | 6.4 g/100 mL (20°C) | Highly soluble (>50 g/100 mL) |
Oxidizing Power | 1.7 V (pH 7) | 1.7 V (pH 7) |
Applications | Water treatment, organic synthesis | Large-scale remediation projects |
Handling | Requires solid dissolution | Ready-to-use liquid form |
Both compounds generate MnO₄⁻ ions, but NaMnO₄ is preferred for subsurface remediation due to its solubility and ease of injection. KMnO₄ is more cost-effective for small-scale applications .
This compound vs. Persulfate
Property | This compound (MnO₄⁻) | Persulfate (S₂O₈²⁻) |
---|---|---|
Redox Potential | 1.7 V (pH 7) | 2.01 V (pH 7) |
Primary Targets | Alkenes, phenols, anilines | Radical-driven oxidation (e.g., via SO₄⁻•) |
Efficiency in PAH Removal | 61–100% (dependent on dose) | ≤39.1% (limited by side reactions) |
Stability | Stable in groundwater | Consumed by non-target organics |
This compound vs. Osmium Tetroxide (OsO₄)
Property | This compound (MnO₄⁻) | Osmium Tetroxide (OsO₄) |
---|---|---|
Oxidation Mechanism | Direct electron transfer | Epoxidation of alkenes |
Toxicity | Low (handled with standard PPE) | Extremely toxic (neurotoxin) |
Applications | Water treatment, microscopy fixation | Organic synthesis, lipid staining |
By-products | MnO₂ (non-toxic precipitate) | Os metal residues (hazardous) |
While both fix biological tissues for microscopy, OsO₄ provides superior membrane contrast but poses significant health risks .
This compound vs. Chlorine and Ozone
Property | This compound (MnO₄⁻) | Chlorine (Cl₂) | Ozone (O₃) |
---|---|---|---|
Redox Potential | 1.7 V | 1.36 V | 2.07 V |
Reaction Rate with Phenolics | Moderate (10⁻²–10⁻³ M⁻¹s⁻¹) | Fast (10³ M⁻¹s⁻¹) | Very fast (10⁵ M⁻¹s⁻¹) |
By-products | MnO₂, CO₂ | Chlorinated organics (e.g., THMs) | Aldehydes, ketones |
This compound’s slower reaction kinetics are offset by its stability in natural waters, avoiding toxic by-products like trihalomethanes (THMs) from chlorine .
Key Research Findings
- Ligand-Enhanced Oxidation: Ligands (e.g., phosphate, humic acid) stabilize Mn(III) intermediates, accelerating this compound’s oxidation of phenolics like bisphenol A (BPA) by up to 10-fold .
- pH Dependence : Alkaline conditions (pH >10) enhance this compound’s efficiency in sulfur oxidation (e.g., converting thiols to sulfonic acids) .
- Microscopy Applications: KMnO₄ fixation preserves membrane structures comparably to OsO₄ but with less toxicity .
Propriétés
Numéro CAS |
14333-13-2 |
---|---|
Formule moléculaire |
MnO4(−) MnO4- |
Poids moléculaire |
118.936 g/mol |
Nom IUPAC |
permanganate |
InChI |
InChI=1S/Mn.4O/q;;;;-1 |
Clé InChI |
NPDODHDPVPPRDJ-UHFFFAOYSA-N |
SMILES |
[O-][Mn](=O)(=O)=O |
SMILES canonique |
[O-][Mn](=O)(=O)=O |
Key on ui other cas no. |
14333-13-2 |
Description physique |
Generally purplish colored. Soluble in water. Noncombustible, but accelerate the burning of combustible material. If the combustible material is finely divided, the mixture may be explosive. May spontaneously ignite in contact with liquid combustible materials. Contact with sulfuric acid may cause fire or explosion. |
Synonymes |
MnO4(1-) MnO4- permanganate permanganic acid |
Origine du produit |
United States |
Avertissement et informations sur les produits de recherche in vitro
Veuillez noter que tous les articles et informations sur les produits présentés sur BenchChem sont destinés uniquement à des fins informatives. Les produits disponibles à l'achat sur BenchChem sont spécifiquement conçus pour des études in vitro, qui sont réalisées en dehors des organismes vivants. Les études in vitro, dérivées du terme latin "in verre", impliquent des expériences réalisées dans des environnements de laboratoire contrôlés à l'aide de cellules ou de tissus. Il est important de noter que ces produits ne sont pas classés comme médicaments et n'ont pas reçu l'approbation de la FDA pour la prévention, le traitement ou la guérison de toute condition médicale, affection ou maladie. Nous devons souligner que toute forme d'introduction corporelle de ces produits chez les humains ou les animaux est strictement interdite par la loi. Il est essentiel de respecter ces directives pour assurer la conformité aux normes légales et éthiques en matière de recherche et d'expérimentation.