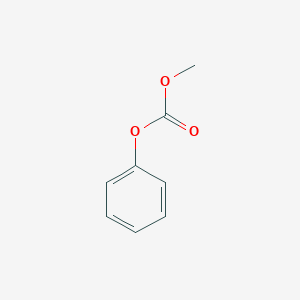
Methyl phenyl carbonate
Vue d'ensemble
Description
Methyl phenyl carbonate is an organic compound with the chemical formula C8H8O3. It is a colorless liquid that is widely used in the chemical and pharmaceutical industries. This compound is known for its versatility and is often employed as a solvent and an intermediate in organic synthesis.
Mécanisme D'action
Target of Action
Methyl phenyl carbonate (MPC) is a chemical compound used widely in the chemical and pharmaceutical industries . Its primary targets are organic solvents, where it acts as a solvent in the manufacture of fragrances, paints, and printing inks .
Mode of Action
MPC interacts with its targets by dissolving or diluting other substances without causing a chemical change to the substance . This property makes it a valuable component in various industrial applications.
Biochemical Pathways
It’s known that mpc is used as a reaction intermediate in the synthesis of other organic compounds, playing a crucial role in organic synthesis .
Result of Action
The result of MPC’s action depends on its application. In its role as a solvent, it helps in the creation of products like fragrances, paints, and printing inks . As a reaction intermediate, it contributes to the synthesis of other organic compounds .
Action Environment
The action, efficacy, and stability of MPC can be influenced by environmental factors. For instance, the reaction of MPC usually requires heating to a reaction temperature (typically between 100-120°C) under alkaline conditions . Furthermore, MPC is a substance with irritant properties and may cause irritation to the skin and eyes . Therefore, appropriate chemical handling and personal protective measures, such as wearing protective clothing and goggles, and ensuring a well-ventilated working environment, should be followed .
Méthodes De Préparation
Synthetic Routes and Reaction Conditions: Methyl phenyl carbonate is typically synthesized through the reaction of methyl formate and phenol under alkaline conditions. The reaction involves mixing methyl formate and phenol, followed by heating the mixture to a temperature range of 100-120°C to facilitate the reaction .
Industrial Production Methods: In industrial settings, the production of this compound often involves the use of catalysts to enhance the reaction efficiency. The process includes steps such as distillation and crystallization to purify the final product .
Analyse Des Réactions Chimiques
Types of Reactions: Methyl phenyl carbonate undergoes various chemical reactions, including:
Oxidation: It can be oxidized to form phenyl carbonate derivatives.
Reduction: Reduction reactions can convert it into phenol and methanol.
Substitution: It can participate in substitution reactions where the methyl or phenyl group is replaced by other functional groups.
Common Reagents and Conditions:
Oxidation: Common oxidizing agents include potassium permanganate and hydrogen peroxide.
Reduction: Reducing agents such as lithium aluminum hydride are often used.
Substitution: Conditions vary depending on the substituent, but typically involve the use of catalysts and specific solvents.
Major Products:
Oxidation: Phenyl carbonate derivatives.
Reduction: Phenol and methanol.
Substitution: Various substituted carbonates depending on the reactants used.
Applications De Recherche Scientifique
Methyl phenyl carbonate has a wide range of applications in scientific research:
Chemistry: It is used as an intermediate in the synthesis of other organic compounds.
Biology: It serves as a reagent in biochemical assays and studies.
Medicine: It is employed in the development of pharmaceuticals and drug delivery systems.
Industry: It is used in the production of polymers, coatings, and adhesives
Comparaison Avec Des Composés Similaires
- Diphenyl carbonate
- Dimethyl carbonate
- Ethyl phenyl carbonate
Comparison: Methyl phenyl carbonate is unique due to its specific reactivity and solubility properties. Unlike diphenyl carbonate, which is more commonly used in the production of polycarbonates, this compound is favored for its use as a solvent and intermediate in organic synthesis. Dimethyl carbonate, on the other hand, is known for its low toxicity and is often used as a green solvent .
Propriétés
IUPAC Name |
methyl phenyl carbonate | |
---|---|---|
Source | PubChem | |
URL | https://pubchem.ncbi.nlm.nih.gov | |
Description | Data deposited in or computed by PubChem | |
InChI |
InChI=1S/C8H8O3/c1-10-8(9)11-7-5-3-2-4-6-7/h2-6H,1H3 | |
Source | PubChem | |
URL | https://pubchem.ncbi.nlm.nih.gov | |
Description | Data deposited in or computed by PubChem | |
InChI Key |
XTBFPVLHGVYOQH-UHFFFAOYSA-N | |
Source | PubChem | |
URL | https://pubchem.ncbi.nlm.nih.gov | |
Description | Data deposited in or computed by PubChem | |
Canonical SMILES |
COC(=O)OC1=CC=CC=C1 | |
Source | PubChem | |
URL | https://pubchem.ncbi.nlm.nih.gov | |
Description | Data deposited in or computed by PubChem | |
Molecular Formula |
C8H8O3 | |
Source | PubChem | |
URL | https://pubchem.ncbi.nlm.nih.gov | |
Description | Data deposited in or computed by PubChem | |
DSSTOX Substance ID |
DTXSID80159247 | |
Record name | Carbonic acid, methyl phenyl ester | |
Source | EPA DSSTox | |
URL | https://comptox.epa.gov/dashboard/DTXSID80159247 | |
Description | DSSTox provides a high quality public chemistry resource for supporting improved predictive toxicology. | |
Molecular Weight |
152.15 g/mol | |
Source | PubChem | |
URL | https://pubchem.ncbi.nlm.nih.gov | |
Description | Data deposited in or computed by PubChem | |
CAS No. |
13509-27-8 | |
Record name | Carbonic acid, methyl phenyl ester | |
Source | ChemIDplus | |
URL | https://pubchem.ncbi.nlm.nih.gov/substance/?source=chemidplus&sourceid=0013509278 | |
Description | ChemIDplus is a free, web search system that provides access to the structure and nomenclature authority files used for the identification of chemical substances cited in National Library of Medicine (NLM) databases, including the TOXNET system. | |
Record name | Carbonic acid, methyl phenyl ester | |
Source | EPA DSSTox | |
URL | https://comptox.epa.gov/dashboard/DTXSID80159247 | |
Description | DSSTox provides a high quality public chemistry resource for supporting improved predictive toxicology. | |
Synthesis routes and methods I
Procedure details
Synthesis routes and methods II
Procedure details
Synthesis routes and methods III
Procedure details
Synthesis routes and methods IV
Procedure details
Retrosynthesis Analysis
AI-Powered Synthesis Planning: Our tool employs the Template_relevance Pistachio, Template_relevance Bkms_metabolic, Template_relevance Pistachio_ringbreaker, Template_relevance Reaxys, Template_relevance Reaxys_biocatalysis model, leveraging a vast database of chemical reactions to predict feasible synthetic routes.
One-Step Synthesis Focus: Specifically designed for one-step synthesis, it provides concise and direct routes for your target compounds, streamlining the synthesis process.
Accurate Predictions: Utilizing the extensive PISTACHIO, BKMS_METABOLIC, PISTACHIO_RINGBREAKER, REAXYS, REAXYS_BIOCATALYSIS database, our tool offers high-accuracy predictions, reflecting the latest in chemical research and data.
Strategy Settings
Precursor scoring | Relevance Heuristic |
---|---|
Min. plausibility | 0.01 |
Model | Template_relevance |
Template Set | Pistachio/Bkms_metabolic/Pistachio_ringbreaker/Reaxys/Reaxys_biocatalysis |
Top-N result to add to graph | 6 |
Feasible Synthetic Routes
Avertissement et informations sur les produits de recherche in vitro
Veuillez noter que tous les articles et informations sur les produits présentés sur BenchChem sont destinés uniquement à des fins informatives. Les produits disponibles à l'achat sur BenchChem sont spécifiquement conçus pour des études in vitro, qui sont réalisées en dehors des organismes vivants. Les études in vitro, dérivées du terme latin "in verre", impliquent des expériences réalisées dans des environnements de laboratoire contrôlés à l'aide de cellules ou de tissus. Il est important de noter que ces produits ne sont pas classés comme médicaments et n'ont pas reçu l'approbation de la FDA pour la prévention, le traitement ou la guérison de toute condition médicale, affection ou maladie. Nous devons souligner que toute forme d'introduction corporelle de ces produits chez les humains ou les animaux est strictement interdite par la loi. Il est essentiel de respecter ces directives pour assurer la conformité aux normes légales et éthiques en matière de recherche et d'expérimentation.