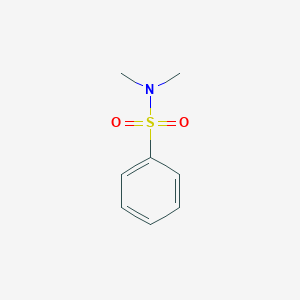
N,N-Dimethylbenzenesulfonamide
Vue d'ensemble
Description
N,N-Dimethylbenzenesulfonamide is an organic compound with the molecular formula C8H11NO2S. It is a sulfonamide derivative where the sulfonamide group is attached to a benzene ring, and the nitrogen atom is substituted with two methyl groups. This compound is known for its applications in various fields, including medicinal chemistry and material science.
Méthodes De Préparation
Synthetic Routes and Reaction Conditions
N,N-Dimethylbenzenesulfonamide can be synthesized through several methods. One common approach involves the reaction of benzenesulfonyl chloride with dimethylamine. The reaction typically occurs in the presence of a base such as triethylamine to neutralize the hydrochloric acid formed during the process. The reaction can be represented as follows:
C6H5SO2Cl+(CH3)2NH→C6H5SO2N(CH3)2+HCl
Industrial Production Methods
In industrial settings, the production of this compound may involve continuous flow processes to enhance efficiency and yield. The use of automated reactors and precise control of reaction parameters such as temperature, pressure, and reactant concentrations can optimize the production process.
Analyse Des Réactions Chimiques
Types of Reactions
N,N-Dimethylbenzenesulfonamide undergoes various chemical reactions, including:
Substitution Reactions: The sulfonamide group can participate in nucleophilic substitution reactions, where the sulfonamide nitrogen can be replaced by other nucleophiles.
Oxidation Reactions: The compound can be oxidized to form sulfone derivatives.
Reduction Reactions: Reduction of the sulfonamide group can lead to the formation of amine derivatives.
Common Reagents and Conditions
Substitution: Reagents such as alkyl halides or acyl chlorides can be used under basic conditions.
Oxidation: Oxidizing agents like hydrogen peroxide or peracids are commonly employed.
Reduction: Reducing agents such as lithium aluminum hydride or catalytic hydrogenation can be used.
Major Products
Substitution: Formation of N-alkyl or N-acyl derivatives.
Oxidation: Formation of sulfone derivatives.
Reduction: Formation of amine derivatives.
Applications De Recherche Scientifique
Medicinal Chemistry
N,N-Dimethylbenzenesulfonamide has gained attention for its potential therapeutic applications, particularly as a sulfonamide antibiotic. Its structural similarity to other sulfonamides allows it to inhibit bacterial dihydropteroate synthase, an enzyme critical for folate synthesis.
Case Study: Antimicrobial Activity
A study evaluated the antimicrobial efficacy of this compound against various bacterial strains. The results indicated significant inhibitory effects on Gram-positive bacteria, highlighting its potential as a lead compound for antibiotic development .
Analytical Chemistry
This compound is utilized as a reagent in various analytical techniques due to its ability to form stable complexes with metal ions. It is used in:
- Chromatography : Employed as a stationary phase modifier in high-performance liquid chromatography (HPLC) to enhance separation efficiency.
- Spectroscopy : Acts as a derivatizing agent in mass spectrometry to improve the detection of polar compounds.
Data Table: Analytical Applications
Biological Studies
This compound is also studied for its effects on cellular processes. Research has shown that it can influence cell signaling pathways and gene expression.
Case Study: Cellular Impact
In vitro studies demonstrated that this compound affects apoptosis pathways in cancer cell lines, suggesting its potential role in cancer therapy .
Environmental Chemistry
The compound's stability and reactivity make it suitable for environmental monitoring applications. It can be used to detect sulfonamide residues in water samples, contributing to studies on water quality and pollution.
Data Table: Environmental Applications
Mécanisme D'action
The mechanism of action of N,N-Dimethylbenzenesulfonamide and its derivatives often involves the inhibition of specific enzymes or receptors. For example, some derivatives are known to inhibit carbonic anhydrase, an enzyme involved in various physiological processes. The inhibition occurs through the binding of the sulfonamide group to the active site of the enzyme, blocking its activity.
Comparaison Avec Des Composés Similaires
Similar Compounds
- N-Methylbenzenesulfonamide
- N,N-Diethylbenzenesulfonamide
- N-Butylbenzenesulfonamide
Uniqueness
N,N-Dimethylbenzenesulfonamide is unique due to its specific substitution pattern, which imparts distinct chemical and biological properties. The presence of two methyl groups on the nitrogen atom enhances its lipophilicity and influences its interaction with biological targets compared to other similar compounds.
Activité Biologique
N,N-Dimethylbenzenesulfonamide (CAS No. 14417-01-7) is a sulfonamide derivative that has garnered attention for its diverse biological activities, particularly in the fields of antimicrobial and antiviral research. This article reviews the biological activity of this compound, highlighting its synthesis, mechanisms of action, and relevant case studies.
Chemical Structure and Properties
This compound has the following chemical structure:
- Molecular Formula : C₈H₁₁N₁O₂S
- Molecular Weight : 185.24 g/mol
- InChI Key : BVSPJPNNLDIUFE-UHFFFAOYSA-N
This compound features a dimethylamino group attached to a benzenesulfonamide moiety, which is critical for its biological activity.
Antimicrobial Activity
Research has demonstrated that this compound exhibits significant antimicrobial properties. A study conducted by Abdel Aal et al. (2023) synthesized various enaminone derivatives conjugated with the dimethylaminosulfonyl moiety and tested their antimicrobial and antifungal activities. The results indicated that several synthesized compounds showed promising activity against various bacterial strains, including Staphylococcus aureus and Escherichia coli .
Table 1: Antimicrobial Activity of this compound Derivatives
Compound ID | Target Organism | Activity (Zone of Inhibition in mm) |
---|---|---|
4a | Staphylococcus aureus | 18 |
4b | Escherichia coli | 15 |
5a | Candida albicans | 20 |
Antiviral Activity
This compound has also been investigated for its antiviral properties. Notably, a sulfanonamide derivative demonstrated high activity against several strains of influenza viruses, including H9N2 and Newcastle disease virus (NDV). The compound exhibited an IC₅₀ value as low as 0.001 mg/mL against H9N2, indicating potent antiviral efficacy .
Case Study: Antiviral Efficacy
In a study published in Molecules, researchers synthesized sulfonamide derivatives and evaluated their antiviral activity against avian paramyxovirus and other viral strains. The findings revealed that modifications to the sulfonamide structure could significantly enhance antiviral potency .
The biological activity of this compound can be attributed to its ability to inhibit key enzymes involved in microbial and viral replication. For instance, sulfonamides generally act by mimicking para-aminobenzoic acid (PABA), a substrate for bacterial folate synthesis, thereby inhibiting bacterial growth .
Molecular Docking Studies
Recent molecular docking studies have provided insights into the binding interactions between this compound derivatives and target proteins associated with cancer cell proliferation. These studies have indicated potential anticancer activity against human liver cancer cell lines, suggesting that these compounds may serve as lead candidates for further development in cancer therapeutics .
Table 2: Molecular Docking Results for Anticancer Activity
Compound ID | Target Protein | Binding Affinity (kcal/mol) |
---|---|---|
4a | EGFR | -9.5 |
4b | VEGFR | -8.7 |
5a | Bcl-2 | -7.8 |
Propriétés
IUPAC Name |
N,N-dimethylbenzenesulfonamide | |
---|---|---|
Source | PubChem | |
URL | https://pubchem.ncbi.nlm.nih.gov | |
Description | Data deposited in or computed by PubChem | |
InChI |
InChI=1S/C8H11NO2S/c1-9(2)12(10,11)8-6-4-3-5-7-8/h3-7H,1-2H3 | |
Source | PubChem | |
URL | https://pubchem.ncbi.nlm.nih.gov | |
Description | Data deposited in or computed by PubChem | |
InChI Key |
BVSPJPNNLDIUFE-UHFFFAOYSA-N | |
Source | PubChem | |
URL | https://pubchem.ncbi.nlm.nih.gov | |
Description | Data deposited in or computed by PubChem | |
Canonical SMILES |
CN(C)S(=O)(=O)C1=CC=CC=C1 | |
Source | PubChem | |
URL | https://pubchem.ncbi.nlm.nih.gov | |
Description | Data deposited in or computed by PubChem | |
Molecular Formula |
C8H11NO2S | |
Source | PubChem | |
URL | https://pubchem.ncbi.nlm.nih.gov | |
Description | Data deposited in or computed by PubChem | |
DSSTOX Substance ID |
DTXSID40162631 | |
Record name | Benzenesulfonamide, N,N-dimethyl- | |
Source | EPA DSSTox | |
URL | https://comptox.epa.gov/dashboard/DTXSID40162631 | |
Description | DSSTox provides a high quality public chemistry resource for supporting improved predictive toxicology. | |
Molecular Weight |
185.25 g/mol | |
Source | PubChem | |
URL | https://pubchem.ncbi.nlm.nih.gov | |
Description | Data deposited in or computed by PubChem | |
CAS No. |
14417-01-7 | |
Record name | N,N-Dimethylbenzenesulfonamide | |
Source | ChemIDplus | |
URL | https://pubchem.ncbi.nlm.nih.gov/substance/?source=chemidplus&sourceid=0014417017 | |
Description | ChemIDplus is a free, web search system that provides access to the structure and nomenclature authority files used for the identification of chemical substances cited in National Library of Medicine (NLM) databases, including the TOXNET system. | |
Record name | N,N-Dimethylbenzenesulfonamide | |
Source | DTP/NCI | |
URL | https://dtp.cancer.gov/dtpstandard/servlet/dwindex?searchtype=NSC&outputformat=html&searchlist=86567 | |
Description | The NCI Development Therapeutics Program (DTP) provides services and resources to the academic and private-sector research communities worldwide to facilitate the discovery and development of new cancer therapeutic agents. | |
Explanation | Unless otherwise indicated, all text within NCI products is free of copyright and may be reused without our permission. Credit the National Cancer Institute as the source. | |
Record name | Benzenesulfonamide, N,N-dimethyl- | |
Source | EPA DSSTox | |
URL | https://comptox.epa.gov/dashboard/DTXSID40162631 | |
Description | DSSTox provides a high quality public chemistry resource for supporting improved predictive toxicology. | |
Record name | N,N-DIMETHYLBENZENESULFONAMIDE | |
Source | FDA Global Substance Registration System (GSRS) | |
URL | https://gsrs.ncats.nih.gov/ginas/app/beta/substances/2K6Y6KZ4UD | |
Description | The FDA Global Substance Registration System (GSRS) enables the efficient and accurate exchange of information on what substances are in regulated products. Instead of relying on names, which vary across regulatory domains, countries, and regions, the GSRS knowledge base makes it possible for substances to be defined by standardized, scientific descriptions. | |
Explanation | Unless otherwise noted, the contents of the FDA website (www.fda.gov), both text and graphics, are not copyrighted. They are in the public domain and may be republished, reprinted and otherwise used freely by anyone without the need to obtain permission from FDA. Credit to the U.S. Food and Drug Administration as the source is appreciated but not required. | |
Synthesis routes and methods I
Procedure details
Synthesis routes and methods II
Procedure details
Retrosynthesis Analysis
AI-Powered Synthesis Planning: Our tool employs the Template_relevance Pistachio, Template_relevance Bkms_metabolic, Template_relevance Pistachio_ringbreaker, Template_relevance Reaxys, Template_relevance Reaxys_biocatalysis model, leveraging a vast database of chemical reactions to predict feasible synthetic routes.
One-Step Synthesis Focus: Specifically designed for one-step synthesis, it provides concise and direct routes for your target compounds, streamlining the synthesis process.
Accurate Predictions: Utilizing the extensive PISTACHIO, BKMS_METABOLIC, PISTACHIO_RINGBREAKER, REAXYS, REAXYS_BIOCATALYSIS database, our tool offers high-accuracy predictions, reflecting the latest in chemical research and data.
Strategy Settings
Precursor scoring | Relevance Heuristic |
---|---|
Min. plausibility | 0.01 |
Model | Template_relevance |
Template Set | Pistachio/Bkms_metabolic/Pistachio_ringbreaker/Reaxys/Reaxys_biocatalysis |
Top-N result to add to graph | 6 |
Feasible Synthetic Routes
Q1: What are some of the synthetic applications of N,N-Dimethylbenzenesulfonamide?
A1: this compound serves as a versatile building block in organic synthesis. For example, it can be ortho-lithiated using n-butyllithium, generating a nucleophilic species. This ortho-lithiosulfonamide reacts with various electrophiles, including benzophenone, benzonitrile, phenylisocyanate, and carbon dioxide, yielding a carbinol, an imine, an amide, and an acid, respectively []. This carbinol can further undergo thermal cyclization to produce a sultone [].
Q2: Can this compound act as an activating group in polymerization reactions?
A2: Yes, research indicates that the sulfonamide moiety in this compound can function as an activating group for nucleophilic aromatic substitution polymerization []. The electron-withdrawing nature of the sulfonamide group activates the aryl fluorides in 2,4-difluoro-N,N-dimethylbenzenesulfonamide, making them susceptible to substitution by phenoxide nucleophiles []. This property enables the synthesis of poly(aryl ether sulfonamide)s [].
Q3: Have any this compound derivatives shown promising antiproliferative activity?
A3: Yes, several pyridine, thiophene, thiazole, chromene, and benzochromene derivatives incorporating the this compound moiety have demonstrated potential as antiproliferative agents []. Notably, compounds incorporating these structures exhibited higher in vitro antiproliferative activity against the MCF-7 human breast cancer cell line than doxorubicin [].
Q4: What is the role of molecular docking studies in understanding the activity of this compound derivatives?
A4: Molecular docking simulations help understand how this compound derivatives interact with potential biological targets. For instance, docking studies have been employed to assess the binding mode of synthesized sulfonamides with carbonic anhydrase IX (CA IX), an enzyme often overexpressed in certain cancer cells []. These insights can guide the design of more potent and selective antiproliferative agents.
Q5: How does the presence of a hydroxyl group influence the hydrolysis of this compound derivatives?
A5: Studies have shown that a neighboring hydroxyl group can significantly impact the hydrolysis rate of this compound derivatives. Specifically, the hydroxyl group participates in intramolecular nucleophilic catalysis during both acid-catalyzed and base-catalyzed hydrolysis of o-(1-hydroxyalkyl)-N,N-dimethylbenzenesulfonamides [, ]. This effect highlights the importance of neighboring group participation in influencing the reactivity of sulfonamide derivatives.
Q6: Can this compound be used to synthesize heterocyclic compounds?
A6: Yes, this compound derivatives can be employed in the synthesis of various heterocyclic systems. For instance, 4-(3-Dimethylaminoacryloyl)-N,N-dimethylbenzenesulfonamide acts as a key intermediate in synthesizing enaminone conjugates with potential biological activity [].
Q7: Are there analytical methods for detecting and quantifying this compound and its derivatives?
A7: Yes, various analytical techniques can be employed to characterize and quantify this compound and its derivatives. Common methods include elemental analysis, infrared spectroscopy (IR), nuclear magnetic resonance spectroscopy (1H-NMR and 13C-NMR), and mass spectrometry [, ]. For instance, enzymatic determination using papain inhibition has been explored for dichlofluanid, a specific this compound derivative [].
Avertissement et informations sur les produits de recherche in vitro
Veuillez noter que tous les articles et informations sur les produits présentés sur BenchChem sont destinés uniquement à des fins informatives. Les produits disponibles à l'achat sur BenchChem sont spécifiquement conçus pour des études in vitro, qui sont réalisées en dehors des organismes vivants. Les études in vitro, dérivées du terme latin "in verre", impliquent des expériences réalisées dans des environnements de laboratoire contrôlés à l'aide de cellules ou de tissus. Il est important de noter que ces produits ne sont pas classés comme médicaments et n'ont pas reçu l'approbation de la FDA pour la prévention, le traitement ou la guérison de toute condition médicale, affection ou maladie. Nous devons souligner que toute forme d'introduction corporelle de ces produits chez les humains ou les animaux est strictement interdite par la loi. Il est essentiel de respecter ces directives pour assurer la conformité aux normes légales et éthiques en matière de recherche et d'expérimentation.