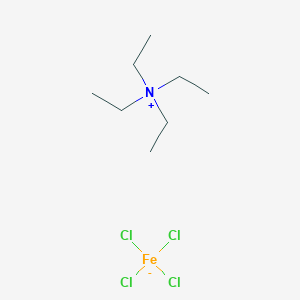
Tetraethylammonium tetrachloroferrate(i&
Vue d'ensemble
Description
Tetraethylammonium tetrachloroferrate(i&, also known as tetraethylammonium tetrachloroferrate(III), is a chemical compound with the molecular formula C₈H₂₀Cl₄FeN and a molecular weight of 327.91 g/mol . This compound is characterized by its yellow to green powder or crystalline appearance and is primarily used in scientific research.
Méthodes De Préparation
The synthesis of tetrachloroiron(1-);tetraethylazanium typically involves the reaction of tetraethylammonium chloride with ferric chloride in an aqueous medium. The reaction is carried out under controlled conditions to ensure the formation of the desired product. The general reaction can be represented as follows:
(C2H5)4NCl+FeCl3→(C2H5)4N[FeCl4]
The product is then isolated and purified through crystallization techniques .
Analyse Des Réactions Chimiques
Tetraethylammonium tetrachloroferrate(i& undergoes various chemical reactions, including:
Oxidation-Reduction Reactions: The iron center in the compound can participate in redox reactions, where it can be reduced or oxidized depending on the reaction conditions.
Substitution Reactions: The chloride ligands in the compound can be substituted by other ligands, such as phosphines or amines, under appropriate conditions.
Coordination Reactions: The compound can form coordination complexes with other metal ions or ligands, leading to the formation of new compounds with different properties.
Applications De Recherche Scientifique
Tetraethylammonium tetrachloroferrate(i& has several applications in scientific research, including:
Catalysis: The compound is used as a catalyst in various organic synthesis reactions, including the formation of carbon-carbon and carbon-heteroatom bonds.
Material Science: It is used in the preparation of advanced materials, such as metal-organic frameworks and coordination polymers.
Electrochemistry: The compound is employed in electrochemical studies to investigate the redox behavior of iron complexes.
Biological Studies: It is used in studies related to the interaction of iron complexes with biological molecules, such as proteins and nucleic acids.
Mécanisme D'action
The mechanism of action of tetrachloroiron(1-);tetraethylazanium involves its ability to interact with various molecular targets, including enzymes and receptors. The iron center in the compound can undergo redox reactions, leading to the generation of reactive oxygen species (ROS) that can affect cellular processes. Additionally, the compound can bind to specific sites on proteins and nucleic acids, modulating their activity and function .
Comparaison Avec Des Composés Similaires
Tetraethylammonium tetrachloroferrate(i& can be compared with other similar compounds, such as:
Tetraethylammonium chloride: This compound is similar in structure but lacks the iron center, making it less versatile in redox and coordination chemistry.
Tetrachloroferrate(III) complexes: These complexes contain the same iron center but differ in the nature of the counterion, which can affect their solubility and reactivity.
Tetraethylammonium bromide and iodide: These compounds are similar in structure but contain different halide ions, which can influence their chemical properties and applications.
This compoundamp; stands out due to its unique combination of tetraethylammonium and tetrachloroferrate(III) moieties, providing distinct chemical and physical properties that make it valuable in various research fields.
Activité Biologique
Tetraethylammonium tetrachloroferrate(III) (TEA-FeCl₄) is an ionic compound that has garnered attention in various fields, including catalysis and biological research. This article explores the biological activity of TEA-FeCl₄, focusing on its antimicrobial properties, potential applications in biomedicine, and relevant research findings.
TEA-FeCl₄ has the molecular formula C₈H₂₀Cl₄FeN and a molecular weight of 327.91 g/mol. The compound is characterized by its ionic nature, where tetraethylammonium acts as the cation and tetrachloroferrate(III) as the anion. Its structure allows for unique interactions with biological molecules, which is pivotal for its biological activity.
Antimicrobial Activity
Research indicates that TEA-FeCl₄ exhibits antimicrobial properties, albeit at moderate concentrations. The mechanism of action is primarily linked to its ability to disrupt microbial cell membranes and interfere with metabolic processes. A study highlighted that the compound functions effectively against various bacterial strains, including Pseudomonas aeruginosa and Klebsiella pneumoniae, which are known for their resistance to conventional antibiotics .
Case Studies
-
Antimicrobial Efficacy :
- A study evaluated the effectiveness of TEA-FeCl₄ against E. coli and Staphylococcus aureus. The results demonstrated a significant reduction in bacterial viability at concentrations above 100 µg/mL.
- The minimal inhibitory concentration (MIC) was determined to be 75 µg/mL for E. coli and 50 µg/mL for S. aureus, indicating its potential as an antimicrobial agent .
- Biofilm Disruption :
Catalytic Applications
In addition to its antimicrobial properties, TEA-FeCl₄ serves as a catalyst in various chemical reactions, particularly in organic synthesis. It has been utilized in the photooxidation of toluene under visible light, demonstrating its versatility beyond biological applications . This catalytic activity is attributed to the dissociation of chlorine atoms from the tetrachloroferrate anion, which can abstract hydrogen from organic substrates .
Research Findings
Recent studies have employed various analytical techniques to characterize TEA-FeCl₄ and assess its biological activity:
- Electrochemical Properties : Investigations into the electrochemical behavior of TEA-FeCl₄ revealed high ionic conductivity, making it suitable for applications in electrochemical sensors and batteries .
- Thermal Stability : Thermal analysis indicated that TEA-FeCl₄ maintains stability up to 200°C before decomposition occurs, which is crucial for storage and application in high-temperature processes .
Data Summary
Property | Value |
---|---|
Molecular Formula | C₈H₂₀Cl₄FeN |
Molecular Weight | 327.91 g/mol |
Antimicrobial Activity (MIC) | 50 µg/mL (S. aureus) |
Biofilm Disruption Efficacy | Effective against P. aeruginosa |
Thermal Stability | Stable up to 200°C |
Propriétés
IUPAC Name |
tetrachloroiron(1-);tetraethylazanium | |
---|---|---|
Source | PubChem | |
URL | https://pubchem.ncbi.nlm.nih.gov | |
Description | Data deposited in or computed by PubChem | |
InChI |
InChI=1S/C8H20N.4ClH.Fe/c1-5-9(6-2,7-3)8-4;;;;;/h5-8H2,1-4H3;4*1H;/q+1;;;;;+3/p-4 | |
Source | PubChem | |
URL | https://pubchem.ncbi.nlm.nih.gov | |
Description | Data deposited in or computed by PubChem | |
InChI Key |
QOQUNYOKJSALRF-UHFFFAOYSA-J | |
Source | PubChem | |
URL | https://pubchem.ncbi.nlm.nih.gov | |
Description | Data deposited in or computed by PubChem | |
Canonical SMILES |
CC[N+](CC)(CC)CC.Cl[Fe-](Cl)(Cl)Cl | |
Source | PubChem | |
URL | https://pubchem.ncbi.nlm.nih.gov | |
Description | Data deposited in or computed by PubChem | |
Molecular Formula |
C8H20Cl4FeN | |
Source | PubChem | |
URL | https://pubchem.ncbi.nlm.nih.gov | |
Description | Data deposited in or computed by PubChem | |
DSSTOX Substance ID |
DTXSID70584327 | |
Record name | Tetraethylammonium tetrachloroferrate | |
Source | EPA DSSTox | |
URL | https://comptox.epa.gov/dashboard/DTXSID70584327 | |
Description | DSSTox provides a high quality public chemistry resource for supporting improved predictive toxicology. | |
Molecular Weight |
327.9 g/mol | |
Source | PubChem | |
URL | https://pubchem.ncbi.nlm.nih.gov | |
Description | Data deposited in or computed by PubChem | |
CAS No. |
14240-75-6 | |
Record name | Tetraethylammonium tetrachloroferrate | |
Source | EPA DSSTox | |
URL | https://comptox.epa.gov/dashboard/DTXSID70584327 | |
Description | DSSTox provides a high quality public chemistry resource for supporting improved predictive toxicology. | |
Record name | Tetraethylammonium iron(III)tetrachloride | |
Source | European Chemicals Agency (ECHA) | |
URL | https://echa.europa.eu/information-on-chemicals | |
Description | The European Chemicals Agency (ECHA) is an agency of the European Union which is the driving force among regulatory authorities in implementing the EU's groundbreaking chemicals legislation for the benefit of human health and the environment as well as for innovation and competitiveness. | |
Explanation | Use of the information, documents and data from the ECHA website is subject to the terms and conditions of this Legal Notice, and subject to other binding limitations provided for under applicable law, the information, documents and data made available on the ECHA website may be reproduced, distributed and/or used, totally or in part, for non-commercial purposes provided that ECHA is acknowledged as the source: "Source: European Chemicals Agency, http://echa.europa.eu/". Such acknowledgement must be included in each copy of the material. ECHA permits and encourages organisations and individuals to create links to the ECHA website under the following cumulative conditions: Links can only be made to webpages that provide a link to the Legal Notice page. | |
Avertissement et informations sur les produits de recherche in vitro
Veuillez noter que tous les articles et informations sur les produits présentés sur BenchChem sont destinés uniquement à des fins informatives. Les produits disponibles à l'achat sur BenchChem sont spécifiquement conçus pour des études in vitro, qui sont réalisées en dehors des organismes vivants. Les études in vitro, dérivées du terme latin "in verre", impliquent des expériences réalisées dans des environnements de laboratoire contrôlés à l'aide de cellules ou de tissus. Il est important de noter que ces produits ne sont pas classés comme médicaments et n'ont pas reçu l'approbation de la FDA pour la prévention, le traitement ou la guérison de toute condition médicale, affection ou maladie. Nous devons souligner que toute forme d'introduction corporelle de ces produits chez les humains ou les animaux est strictement interdite par la loi. Il est essentiel de respecter ces directives pour assurer la conformité aux normes légales et éthiques en matière de recherche et d'expérimentation.