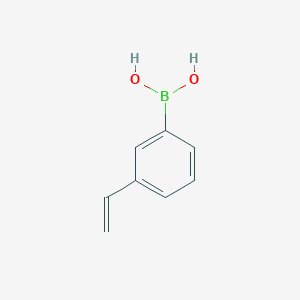
Acide 3-vinylphénylboronique
Vue d'ensemble
Description
3-Vinylphenylboronic acid, also known as (3-Ethenylphenyl)boronic acid or m-Vinylbenzeneboronic acid, is an organoboron compound with the molecular formula C8H9BO2. It is a white to off-white crystalline powder that is soluble in organic solvents. This compound is widely used in organic synthesis, particularly in Suzuki-Miyaura cross-coupling reactions, due to its ability to form carbon-carbon bonds.
Applications De Recherche Scientifique
3-Vinylphenylboronic acid has a wide range of applications in scientific research:
Mécanisme D'action
Target of Action
3-Vinylphenylboronic acid is a reactant involved in various chemical reactions . It is primarily used in Suzuki-Miyaura reactions , a type of cross-coupling reaction, where it acts as a boron-based reagent . It also plays a role in the molecular imprinting of fructose and pinacol , and the synthesis of arylmercapturic acids via Chen-Lam-Evans arylation .
Mode of Action
The compound interacts with its targets through covalent bonding, forming new compounds in the process . For instance, in Suzuki-Miyaura reactions, it forms a complex with a palladium catalyst and undergoes a transmetallation process, resulting in the formation of new carbon-carbon bonds .
Biochemical Pathways
It is known to be involved in the generation of acyl anion equivalents and three-component cross-coupling yielding diarylketones . These reactions are crucial in organic synthesis, leading to the formation of complex organic compounds.
Pharmacokinetics
It is known that the compound has high gi absorption . The compound’s ADME (Absorption, Distribution, Metabolism, and Excretion) properties and their impact on bioavailability remain to be explored.
Result of Action
The primary result of 3-Vinylphenylboronic acid’s action is the formation of new organic compounds through various chemical reactions . For instance, in Suzuki-Miyaura reactions, it helps in the formation of biaryl compounds, which are important in pharmaceuticals and materials science .
Action Environment
The action of 3-Vinylphenylboronic acid can be influenced by environmental factors such as temperature and pH. It is typically stored at temperatures between 2-8°C . The compound may contain varying amounts of anhydride, which could potentially affect its reactivity
Méthodes De Préparation
Synthetic Routes and Reaction Conditions: The primary method for synthesizing 3-Vinylphenylboronic acid involves the electrophilic trapping of an organometallic reagent with a boric ester, such as triisopropyl borate or trimethyl borate. The reaction is typically performed at low temperatures to prevent over-alkylation, which could lead to the formation of borinic esters instead of boronic esters .
Industrial Production Methods: In industrial settings, the synthesis of 3-Vinylphenylboronic acid can be scaled up using similar methods. The process involves the careful control of reaction conditions, such as temperature and reagent concentrations, to ensure high yields and purity. The product is then purified through recrystallization or chromatography techniques.
Analyse Des Réactions Chimiques
Types of Reactions: 3-Vinylphenylboronic acid undergoes various chemical reactions, including:
Suzuki-Miyaura Cross-Coupling: This reaction involves the coupling of 3-Vinylphenylboronic acid with halides or triflates in the presence of a palladium catalyst and a base.
Oxidation: The compound can be oxidized to form phenols or quinones under specific conditions.
Substitution: It can participate in electrophilic aromatic substitution reactions, where the vinyl group can be further functionalized.
Common Reagents and Conditions:
Suzuki-Miyaura Cross-Coupling: Palladium catalysts (e.g., Pd(PPh3)4), bases (e.g., potassium carbonate), and solvents (e.g., toluene or ethanol) are commonly used.
Oxidation: Oxidizing agents such as hydrogen peroxide or potassium permanganate can be used.
Substitution: Electrophiles such as halogens or nitro groups can be introduced under acidic or basic conditions.
Major Products Formed:
Biaryl Compounds: Formed through Suzuki-Miyaura cross-coupling.
Phenols and Quinones: Formed through oxidation reactions.
Functionalized Aromatics: Formed through substitution reactions.
Comparaison Avec Des Composés Similaires
Phenylboronic Acid: Similar structure but lacks the vinyl group.
4-Vinylphenylboronic Acid: Similar structure with the vinyl group in the para position.
2-Methoxy-3-pyridinylboronic Acid: Contains a pyridine ring and a methoxy group, offering different reactivity and applications.
Uniqueness: 3-Vinylphenylboronic acid is unique due to the position of the vinyl group, which influences its reactivity and selectivity in chemical reactions. This makes it particularly useful in the synthesis of specific biaryl compounds and in applications where the electronic properties of the vinyl group are advantageous .
Propriétés
IUPAC Name |
(3-ethenylphenyl)boronic acid | |
---|---|---|
Source | PubChem | |
URL | https://pubchem.ncbi.nlm.nih.gov | |
Description | Data deposited in or computed by PubChem | |
InChI |
InChI=1S/C8H9BO2/c1-2-7-4-3-5-8(6-7)9(10)11/h2-6,10-11H,1H2 | |
Source | PubChem | |
URL | https://pubchem.ncbi.nlm.nih.gov | |
Description | Data deposited in or computed by PubChem | |
InChI Key |
SYBQEKBVWDPVJM-UHFFFAOYSA-N | |
Source | PubChem | |
URL | https://pubchem.ncbi.nlm.nih.gov | |
Description | Data deposited in or computed by PubChem | |
Canonical SMILES |
B(C1=CC(=CC=C1)C=C)(O)O | |
Source | PubChem | |
URL | https://pubchem.ncbi.nlm.nih.gov | |
Description | Data deposited in or computed by PubChem | |
Molecular Formula |
C8H9BO2 | |
Source | PubChem | |
URL | https://pubchem.ncbi.nlm.nih.gov | |
Description | Data deposited in or computed by PubChem | |
DSSTOX Substance ID |
DTXSID30402437 | |
Record name | 3-Vinylphenylboronic acid | |
Source | EPA DSSTox | |
URL | https://comptox.epa.gov/dashboard/DTXSID30402437 | |
Description | DSSTox provides a high quality public chemistry resource for supporting improved predictive toxicology. | |
Molecular Weight |
147.97 g/mol | |
Source | PubChem | |
URL | https://pubchem.ncbi.nlm.nih.gov | |
Description | Data deposited in or computed by PubChem | |
CAS No. |
15016-43-0 | |
Record name | (3-Vinylphenyl)boronic acid | |
Source | CAS Common Chemistry | |
URL | https://commonchemistry.cas.org/detail?cas_rn=15016-43-0 | |
Description | CAS Common Chemistry is an open community resource for accessing chemical information. Nearly 500,000 chemical substances from CAS REGISTRY cover areas of community interest, including common and frequently regulated chemicals, and those relevant to high school and undergraduate chemistry classes. This chemical information, curated by our expert scientists, is provided in alignment with our mission as a division of the American Chemical Society. | |
Explanation | The data from CAS Common Chemistry is provided under a CC-BY-NC 4.0 license, unless otherwise stated. | |
Record name | 3-Vinylphenylboronic acid | |
Source | EPA DSSTox | |
URL | https://comptox.epa.gov/dashboard/DTXSID30402437 | |
Description | DSSTox provides a high quality public chemistry resource for supporting improved predictive toxicology. | |
Retrosynthesis Analysis
AI-Powered Synthesis Planning: Our tool employs the Template_relevance Pistachio, Template_relevance Bkms_metabolic, Template_relevance Pistachio_ringbreaker, Template_relevance Reaxys, Template_relevance Reaxys_biocatalysis model, leveraging a vast database of chemical reactions to predict feasible synthetic routes.
One-Step Synthesis Focus: Specifically designed for one-step synthesis, it provides concise and direct routes for your target compounds, streamlining the synthesis process.
Accurate Predictions: Utilizing the extensive PISTACHIO, BKMS_METABOLIC, PISTACHIO_RINGBREAKER, REAXYS, REAXYS_BIOCATALYSIS database, our tool offers high-accuracy predictions, reflecting the latest in chemical research and data.
Strategy Settings
Precursor scoring | Relevance Heuristic |
---|---|
Min. plausibility | 0.01 |
Model | Template_relevance |
Template Set | Pistachio/Bkms_metabolic/Pistachio_ringbreaker/Reaxys/Reaxys_biocatalysis |
Top-N result to add to graph | 6 |
Feasible Synthetic Routes
Q1: How does 3-Vinylphenylboronic acid contribute to the unique properties of the boroxine-based thermosets described in the research?
A1: 3-Vinylphenylboronic acid plays a crucial role as a building block in the synthesis of these innovative thermosets. [] It functions as a monomer, alongside other monomers like 4-vinylphenylboronic acid and octadecanoxy polyethylene glycol methacrylate, to form random copolymers. [] The key feature of 3-Vinylphenylboronic acid is its boronic acid group. This group enables the formation of reversible boroxine bonds during the curing process, which are responsible for several remarkable properties of the resulting thermosets: []
Q2: Are there any differences in the properties of the thermosets when using 3-Vinylphenylboronic acid compared to its isomer, 4-Vinylphenylboronic acid, in the synthesis?
A2: While the research primarily focuses on 4-Vinylphenylboronic acid for tailoring the tensile strength of the thermosets, the use of 3-Vinylphenylboronic acid, as an alternative isomer, could potentially lead to variations in material properties. [] Further research is needed to investigate:
Avertissement et informations sur les produits de recherche in vitro
Veuillez noter que tous les articles et informations sur les produits présentés sur BenchChem sont destinés uniquement à des fins informatives. Les produits disponibles à l'achat sur BenchChem sont spécifiquement conçus pour des études in vitro, qui sont réalisées en dehors des organismes vivants. Les études in vitro, dérivées du terme latin "in verre", impliquent des expériences réalisées dans des environnements de laboratoire contrôlés à l'aide de cellules ou de tissus. Il est important de noter que ces produits ne sont pas classés comme médicaments et n'ont pas reçu l'approbation de la FDA pour la prévention, le traitement ou la guérison de toute condition médicale, affection ou maladie. Nous devons souligner que toute forme d'introduction corporelle de ces produits chez les humains ou les animaux est strictement interdite par la loi. Il est essentiel de respecter ces directives pour assurer la conformité aux normes légales et éthiques en matière de recherche et d'expérimentation.