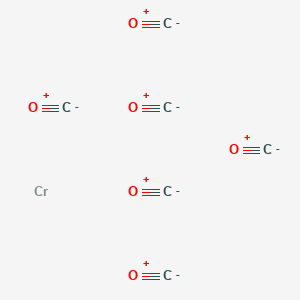
Chromium(0) hexacarbonyl
Vue d'ensemble
Description
Chromium(0) hexacarbonyl, Cr(CO)₆, is a homoleptic organometallic compound in which a chromium atom in the zero oxidation state is coordinated by six carbon monoxide ligands in an octahedral geometry . This air-stable, diamagnetic solid appears as white to yellow crystals or powder with a density of 1.77 g/cm³ and a melting point exceeding 150°C (decomposition) . Its synthesis typically involves the reaction of chromium salts with carbon monoxide under high pressure or photolytic substitution of CO ligands in coordinating solvents like tetrahydrofuran (THF) .
Cr(CO)₆ serves as a versatile precursor in organic and inorganic chemistry, enabling the synthesis of chromium-containing catalysts, advanced materials for electronics, and ligands for transition metal complexes . Notably, its photochemical reactivity facilitates ligand substitution, making it valuable in depositing chromium layers for nanofabrication . However, it is classified as hazardous (H301: toxic if swallowed) and requires careful handling .
Méthodes De Préparation
Chromium(0) hexacarbonyl is typically prepared through a process known as “reductive carbonylation.” This involves the reduction of a metal halide under an atmosphere of carbon monoxide. The most cost-effective routes for synthesizing group 6 hexacarbonyls, including chromium carbonyl, involve the reduction of metal chlorides (such as CrCl3) with magnesium, zinc, or aluminum powders under carbon monoxide pressures . Another method involves the direct reaction of specially produced chromium metal with carbon monoxide gas, although this method is not commonly used commercially .
Analyse Des Réactions Chimiques
Substitution Reactions
Cr(CO)₆ undergoes ligand substitution, where CO ligands are replaced by stronger field ligands. A notable example involves cyclopentadienyl (Cp) transfer:
Reaction Type | Example | Product | Source |
---|---|---|---|
Ligand substitution | Cr(CO)₆ + KReO₄ → Re(CO)₃(C₅H₅) + ... | Re(CO)₃(C₅H₅) | |
Carbonyl displacement | Cr(CO)₆ + PR₃ → Cr(CO)₅(PR₃) + CO | Phosphine-substituted Cr(0) |
Such reactions exploit the labile nature of CO ligands under thermal or photolytic conditions.
Redox Reactivity
Cr(CO)₆ participates in redox processes, acting as a reducing agent due to its low oxidation state:
Reaction Conditions | Outcome | Oxidation State Change | Source |
---|---|---|---|
Reaction with HCl | Cr(CO)₆ + HCl → CrCl₂/CrCl₃ + CO + H₂ | Cr⁰ → Cr²⁺/Cr³⁺ | |
Oxidative decarbonylation | Cr(CO)₆ + O₂ → CrO₃ + CO₂ | Cr⁰ → Cr⁶⁺ |
These reactions highlight chromium’s ability to adopt oxidation states from 0 to +6 .
Dissociative Electron Attachment (DEA) Studies
Gas-phase DEA experiments reveal fragmentation patterns under electron bombardment :
Fragment Anion | Peak Energy (eV) | Proposed Mechanism |
---|---|---|
[Cr(CO)₅]⁻ | 0–1.5 | Loss of one CO ligand |
[Cr(CO)₄]⁻ | 1.4, 4.1 | Sequential CO loss via 3t₂u orbital resonance |
[Cr(CO)₃]⁻ | 4.5 | Further decarbonylation |
DEA results indicate Cr–CO bond dissociation energies of ~1.6 eV for [Cr(CO)₅]⁻ formation . Comparative studies with (η⁶-C₆H₆)Cr(CO)₃ show stronger Cr–CO bonds in heteroleptic complexes .
Comparative Stability and Bonding
The Dewar-Chatt-Duncanson model explains Cr(CO)₆’s stability through synergistic σ-donation and π-backbonding. Key bond metrics :
Parameter | Value (Å) | Method/Source |
---|---|---|
Cr–C bond length | 1.916 | X-ray crystallography |
C–O bond length | 1.171 | X-ray crystallography |
HOMO-LUMO gap | Calculated | DFT studies |
Applications De Recherche Scientifique
Preparation Methods
Chromium(0) hexacarbonyl is synthesized through reductive carbonylation, typically involving the reduction of chromium halides under carbon monoxide atmosphere. Common reducing agents include magnesium, zinc, or aluminum powders.
Catalysis
- Olefin Polymerization and Isomerization : this compound acts as a catalyst in the polymerization of olefins, enhancing the efficiency of these reactions and leading to higher yields of desired products .
- Fischer-Tropsch Synthesis : It serves as a precursor for Fischer carbenes, which are crucial in organic synthesis and catalysis .
Thin Film Deposition
- Chemical Vapor Deposition (CVD) : this compound is used in CVD processes to produce thin films of chromium. This application is significant in electronics and materials science for creating coatings that enhance surface properties .
- Atomic Layer Deposition (ALD) : The compound's volatility and stability make it suitable for ALD techniques, allowing precise control over film thickness at low temperatures .
Material Science
- Corrosion-Resistant Coatings : Chromium carbonyl complexes are employed to develop coatings that provide resistance to corrosion, making them valuable in various industrial applications .
- Abrasive Metallurgy : It is used as an active filler in abrasive materials due to its hardness and stability.
Biological Applications
- Medicinal Chemistry : Research has explored chromium carbonyl complexes for their potential therapeutic effects, including anti-cancer properties. These studies focus on their interaction with biological systems and potential drug development .
Environmental Applications
- Photocatalysis : this compound has demonstrated photocatalytic activity in reactions such as hydrosilylation, which can be utilized for environmental remediation processes .
Case Study 1: Photochemical Reactions
Research has shown that upon UV irradiation in tetrahydrofuran (THF), this compound can convert to Cr(CO)₅(THF), demonstrating its utility in photochemical applications. This transformation allows for the generation of labile complexes that can be further utilized in synthetic pathways .
Case Study 2: Olefin Polymerization
In a study examining the efficiency of chromium-based catalysts for olefin polymerization, this compound was found to significantly enhance reaction rates compared to traditional catalysts, leading to higher molecular weight polymers with improved mechanical properties .
Mécanisme D'action
The mechanism by which chromium carbonyl exerts its effects involves the coordination of carbon monoxide ligands to the central chromium atom. This coordination results in the formation of a stable octahedral complex. The carbon monoxide ligands can engage in π-backbonding with the chromium atom, which stabilizes the complex and influences its reactivity . The molecular targets and pathways involved in the action of chromium carbonyl depend on the specific reactions and applications in which it is used.
Comparaison Avec Des Composés Similaires
Comparison with Similar Chromium Carbonyl Complexes
Homoleptic vs. Heteroleptic Chromium Complexes
Cr(CO)₆ is homoleptic, whereas complexes like benzene-chromium(0) tricarbonyl, (η⁶-C₆H₆)Cr(CO)₃, are heteroleptic, combining CO and arene ligands. The substitution of three CO ligands with a benzene ring in (η⁶-C₆H₆)Cr(CO)₃ alters its geometry to a "piano stool" structure, impacting stability and reactivity . For example, (η⁶-C₆H₆)Cr(CO)₃ exhibits enhanced electron-donating properties due to the benzene ligand, making it more reactive in stoichiometric substitution reactions compared to Cr(CO)₆ .
Ligand Substitution and Stability
Bis(benzene)chromium(0), (η⁶-C₆H₆)₂Cr, lacks CO ligands and is highly susceptible to oxidation, unlike Cr(CO)₆, which is air-stable . Mixed-ligand complexes like (η⁶-C₆H₆)Cr(CO)₃ can be synthesized via CO displacement from Cr(CO)₆ under UV irradiation, demonstrating the labile nature of CO ligands in photochemical reactions .
Reactivity in Electron Attachment Studies
Dissociative electron attachment (DEA) studies reveal distinct fragmentation patterns: Cr(CO)₆ generates [Cr(CO)₅]⁻, [Cr(CO)₄]⁻, and [Cr(CO)₃]⁻ ions, while (η⁶-C₆H₆)Cr(CO)₃ produces seven fragment ions, including [Cr(C₆H₆)(CO)₂]⁻ and [Cr]⁻. This highlights the stronger bonding of benzene ligands compared to CO in heteroleptic complexes .
Comparison with Other Metal Carbonyls
Rhodium Hexadecacarbonyl (Rh₆(CO)₁₆)
Rh₆(CO)₁₆ features a hexarhodium core with 16 CO ligands, contrasting with Cr(CO)₆'s mononuclear structure. Rhodium carbonyls are used in catalysis for hydroformylation, whereas Cr(CO)₆ is employed in polymerization and nanomaterial synthesis .
Iron Pentacarbonyl (Fe(CO)₅)
Fe(CO)₅ is a liquid under standard conditions (melting point: -20°C), unlike solid Cr(CO)₆. Both are homoleptic, but Fe(CO)₅ is more volatile and toxic, releasing CO gas upon decomposition .
Data Tables
Table 1: Comparison of Chromium Carbonyl Complexes
Table 2: Comparison with Other Metal Carbonyls
Property | Cr(CO)₆ | Rh₆(CO)₁₆ | Fe(CO)₅ |
---|---|---|---|
Metal Oxidation State | 0 | 0 | 0 |
Physical State | Solid | Solid | Liquid |
Melting Point | >150°C (dec.) | >200°C | -20°C |
Key Use | Nanomaterial deposition | Hydroformylation | CO gas generation |
Toxicity | H301 (oral) | High | High (CO release) |
Reference |
Activité Biologique
Chromium(0) hexacarbonyl (Cr(CO)₆) is a homoleptic organometallic compound with significant biochemical implications. Its biological activity is primarily linked to its interactions with cellular components, enzymatic pathways, and potential toxicological effects. This article delves into the mechanisms of action, biochemical pathways, and relevant case studies that illustrate the compound's biological significance.
This compound is characterized by its six carbon monoxide ligands coordinated to a chromium atom in the zero oxidation state. The molecular structure features Cr–C and C–O bond lengths of approximately 1.916 Å and 1.171 Å, respectively . This configuration contributes to its stability and volatility, making it a subject of interest in both industrial applications and biological research.
Target Interactions
this compound primarily interacts with various biomolecules, including proteins and nucleic acids. It has been shown to bind with cytochrome P450 enzymes, which are crucial for drug metabolism and synthesis of steroid hormones. This interaction can lead to the formation of reactive intermediates that may damage cellular components.
Biochemical Pathways
The compound influences several biochemical pathways:
- Oxidation of Alcohols : Cr(CO)₆ facilitates the conversion of alcohols into carbonyl compounds through enzymatic reactions, impacting metabolic processes.
- Oxidative Stress Induction : Chromium carbonyl can induce oxidative stress in cells, activating stress response pathways that may lead to cell damage or apoptosis .
- Inhibition of Key Enzymes : It can inhibit metabolic enzymes involved in critical pathways such as the citric acid cycle, affecting energy production within cells.
Pharmacokinetics
The pharmacokinetics of chromium carbonyl involves:
- Absorption and Distribution : Upon entering biological systems, it can localize in cellular compartments like mitochondria and nuclei, where it exerts its effects.
- Transport Mechanisms : It binds to serum albumin and other plasma proteins, facilitating its transport in the bloodstream.
Toxicological Profile
This compound exhibits toxicity that varies with dosage:
- Low Doses : Minimal impact on cellular function.
- High Doses : Significant toxicity leading to cellular dysfunction and death due to oxidative stress and enzyme inhibition .
Case Studies
Several studies have highlighted the toxicological effects of chromium carbonyl:
- In Vivo Studies : Animal models exposed to chromium carbonyl showed dose-dependent toxicity, with higher concentrations leading to liver and kidney damage due to oxidative stress .
- Cell Culture Experiments : In vitro studies demonstrated that chromium carbonyl exposure resulted in increased reactive oxygen species (ROS) production, leading to DNA damage and altered gene expression profiles in cultured cells .
Data Table: Biological Effects of this compound
Q & A
Basic Research Questions
Q. What are the established methods for synthesizing chromium(0) hexacarbonyl, and how can experimental parameters influence yield and purity?
this compound (Cr(CO)₆) is typically synthesized via the direct reaction of chromium metal with carbon monoxide under high-pressure conditions. However, recent advancements employ redox reactions using strong oxidizing agents like [NO]⁺ paired with weakly coordinating anions (e.g., [Al(ORF)₄]⁻) to stabilize reactive intermediates. For example, oxidation of Cr(CO)₆ with [NO][Al(ORF)₄] in nonpolar solvents (e.g., CH₂Cl₂) under inert atmospheres (Schlenk techniques) yields stable cationic species like [Cr(CO)₆]⁺ salts at room temperature . Key parameters include:
- Solvent choice : Polar solvents may destabilize intermediates, while nonpolar solvents enhance crystallinity.
- Temperature : Reactions conducted below 0°C reduce ligand dissociation.
- Anion selection : Weakly coordinating anions (e.g., [Al(ORF)₄]⁻) prevent unwanted ion pairing.
Q. How can the octahedral geometry and electronic structure of Cr(CO)₆ be experimentally validated?
The octahedral geometry of Cr(CO)₆ is confirmed via:
- X-ray crystallography : Bond lengths (Cr–C: 1.91 Å, C–O: 1.14 Å) and Oh symmetry align with DFT calculations .
- Infrared spectroscopy : CO stretching vibrations (νCO ≈ 2000 cm⁻¹) indicate strong backbonding from Cr(0) to CO ligands .
- Mass spectrometry : Parent ion peaks (m/z ≈ 220) and fragmentation patterns (e.g., loss of CO ligands) validate molecular stability .
Q. What precautions are critical for handling Cr(CO)₆ in laboratory settings?
Cr(CO)₆ is light-sensitive and volatile. Best practices include:
- Storage : Inert atmosphere (Ar/N₂) at room temperature, shielded from UV light .
- Glovebox/Schlenk use : Prevents oxidation and ligand loss during synthesis .
- Ventilation : Local exhaust systems mitigate inhalation risks due to sublimation .
Advanced Research Questions
Q. How does dissociative electron attachment (DEA) to Cr(CO)₆ influence its fragmentation pathways, and what insights does this provide for nanofabrication?
DEA studies reveal Cr(CO)₆ fragmentation into anions like [Cr(CO)₅]⁻, [Cr(CO)₄]⁻, and [Cr(CO)₃]⁻ via low-energy electron interactions (0–12 eV). Key findings:
- Fragment ion yields : Dominant [Cr(CO)₅]⁻ formation (0–1.5 eV) suggests CO ligand loss via transient negative ion states .
- Energy dependence : Higher-energy resonances (4–8 eV) induce sequential CO dissociation (Table 1).
Fragment Anion | Resonance Energy (eV) | Relative Intensity |
---|---|---|
[Cr(CO)₅]⁻ | 0–1.5 | High |
[Cr(CO)₄]⁻ | 1.4–4.0 | Moderate |
[Cr(CO)₃]⁻ | 4.7–8.0 | Low |
These results inform focused electron beam-induced deposition (FEBID) for Cr-based nanostructures, where ligand stability impacts carbon contamination .
Q. What challenges arise in preparing stable chromium(I) hexacarbonyl cations, and how can they be addressed methodologically?
The [Cr(CO)₆]⁺ cation is highly reactive due to its 17-electron configuration. Stabilization strategies include:
- Oxidant selection : [NO]⁺ avoids nitrosylation side reactions seen with stronger oxidants .
- Counterion pairing : Bulky anions (e.g., [Al(ORF)₄]⁻) reduce ion pairing and enhance solubility .
- Crystallization conditions : Slow diffusion of hexane into CH₂Cl₂ solutions yields X-ray-quality crystals .
Q. How do ligand substitution reactions in Cr(CO)₆ enable the synthesis of heteroleptic complexes, and what analytical methods track these transformations?
Substitution of CO ligands with π-donors (e.g., benzene) proceeds via photolytic or thermal activation:
- Mechanism : UV irradiation (λ = 350 nm) cleaves Cr–CO bonds, enabling benzene (η⁶-C₆H₆) coordination to form (η⁶-C₆H₆)Cr(CO)₃ .
- Monitoring :
Q. What thermodynamic data are essential for predicting Cr(CO)₆ decomposition under varying conditions?
Critical parameters include:
- Vapor pressure : ~1.77 g/mL at 25°C, requiring sublimation traps in vacuum systems .
- Thermal stability : Decomposes above 150°C, releasing CO and forming Cr metal (ΔrH° = +210 kJ/mol) .
- Electron impact cross-sections : Quantify DEA efficiency for nanofabrication applications .
Q. Methodological Guidance
- Contradiction resolution : Conflicting DEA fragment intensities (e.g., [Cr(CO)₅]⁻ vs. [Cr(CO)₃]⁻) may arise from electron energy calibration errors. Cross-validate using tandem MS/MS and theoretical calculations .
- Data reproducibility : Document Schlenk line oxygen/moisture levels (<1 ppm) to ensure synthesis consistency .
Propriétés
Numéro CAS |
13007-92-6 |
---|---|
Formule moléculaire |
C6CrO6 |
Poids moléculaire |
220.06 g/mol |
Nom IUPAC |
carbon monoxide;chromium |
InChI |
InChI=1S/6CO.Cr/c6*1-2; |
Clé InChI |
KOTQLLUQLXWWDK-UHFFFAOYSA-N |
SMILES |
[C-]#[O+].[C-]#[O+].[C-]#[O+].[C-]#[O+].[C-]#[O+].[C-]#[O+].[Cr] |
SMILES canonique |
[C-]#[O+].[C-]#[O+].[C-]#[O+].[C-]#[O+].[C-]#[O+].[C-]#[O+].[Cr] |
Point d'ébullition |
410 °F at 760 mm Hg (violent decomposition) (NTP, 1992) |
Densité |
1.77 at 64 °F (NTP, 1992) |
melting_point |
230 °F (decomposes) (NTP, 1992) |
Key on ui other cas no. |
13007-92-6 |
Description physique |
Chromium carbonyl appears as white crystalline or granular solid. Sublimes at room temperature. Burns with a luminous flame. (NTP, 1992) |
Pictogrammes |
Acute Toxic |
Solubilité |
less than 1 mg/mL at 68° F (NTP, 1992) |
Synonymes |
chromium carbonyl chromium hexacarbonyl |
Pression de vapeur |
1 mm Hg at 97 °F ; 760 mm Hg at 304° F (NTP, 1992) |
Origine du produit |
United States |
Synthesis routes and methods
Procedure details
Avertissement et informations sur les produits de recherche in vitro
Veuillez noter que tous les articles et informations sur les produits présentés sur BenchChem sont destinés uniquement à des fins informatives. Les produits disponibles à l'achat sur BenchChem sont spécifiquement conçus pour des études in vitro, qui sont réalisées en dehors des organismes vivants. Les études in vitro, dérivées du terme latin "in verre", impliquent des expériences réalisées dans des environnements de laboratoire contrôlés à l'aide de cellules ou de tissus. Il est important de noter que ces produits ne sont pas classés comme médicaments et n'ont pas reçu l'approbation de la FDA pour la prévention, le traitement ou la guérison de toute condition médicale, affection ou maladie. Nous devons souligner que toute forme d'introduction corporelle de ces produits chez les humains ou les animaux est strictement interdite par la loi. Il est essentiel de respecter ces directives pour assurer la conformité aux normes légales et éthiques en matière de recherche et d'expérimentation.