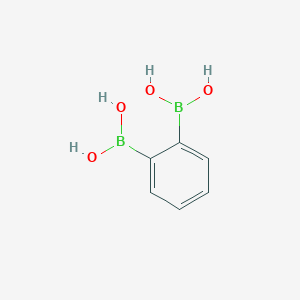
1,2-Phenylenediboronic acid
Vue d'ensemble
Description
1,2-Phenylenediboronic acid is a compound that is generally used in metal-catalyzed C-C bond formation reactions . It is also used for palladium-catalyzed sequential alkenylation and conjugate addition reactions, scholl cyclizations, Suzuki-Miyaura cross-coupling reactions .
Synthesis Analysis
1,2-Phenylenediboronic esters have been explored as potential bidentate catalysts for organic synthesis . The synthesis of these esters relied on tetrachloro-1,2-bisborobenzene as a synthetic intermediate, which can be obtained by metal exchange from the corresponding 1,2-bis(trimethylsilyl)benzene .Molecular Structure Analysis
The molecular formula of 1,2-Phenylenediboronic acid is C6H8B2O4 . The InChI code is 1S/C6H8B2O4/c9-7(10)5-3-1-2-4-6(5)8(11)12/h1-4,9-12H .Chemical Reactions Analysis
1,2-Phenylenediboronic acid has been used in the preparation of indolizine derivatives as OLEDs, in organic thin-film transistors, and in fluorescence and solution-processable coordination polymers . It has also been used in the synthesis of organogels by reaction with a diol-containing polyether .Physical And Chemical Properties Analysis
The molecular weight of 1,2-Phenylenediboronic acid is 165.75 g/mol . It has a hydrogen bond donor count of 4 and a hydrogen bond acceptor count of 4 . The exact mass is 166.0608691 g/mol .Applications De Recherche Scientifique
KPC/AmpC Inhibitors
1,2-Phenylenediboronic acid has been investigated as a potential inhibitor of KPC/AmpC, which are types of β-lactamase enzymes . The compound was found to reduce the minimum inhibitory concentrations (MICs) of carbapenems, a class of antibiotics, by up to 16/8-fold at a concentration of 8/4 mg/L . This suggests that 1,2-Phenylenediboronic acid could be a promising scaffold for the future development of novel KPC/AmpC inhibitors .
Organic Synthesis Catalyst
1,2-Phenylenediboronic esters, derivatives of 1,2-Phenylenediboronic acid, have been explored as potential bidentate catalysts for organic synthesis . They offer higher stability and easier handling than the currently used boranthracene derivatives . These esters have been particularly investigated for their ability to catalyze the inverse electron demand Diels–Alder reaction of 1,2-diazene .
Bioconjugate Preparation
1,2-Phenylenediboronic acid has been used in the field of bioconjugate preparation . Specifically, it has been used to create a novel class of reagents useful for the conjugation of biological macromolecules .
Energy Transfer Processes in Optoelectronic Devices
1,2-Phenylenediboronic acid has been used in energy transfer processes in optoelectronic devices . The specific details of its application in this field are not readily available, but it’s an area of ongoing research.
Palladium-Catalyzed Sequential Alkenylation and Conjugate Addition Reactions
1,2-Phenylenediboronic acid has been used in palladium-catalyzed sequential alkenylation and conjugate addition reactions . These reactions are important in the synthesis of complex organic molecules.
Scholl Cyclizations
1,2-Phenylenediboronic acid has been used in Scholl cyclizations , a type of chemical reaction used in the synthesis of polycyclic aromatic compounds.
Mécanisme D'action
Target of Action
1,2-Phenylenediboronic acid has been investigated as a potential catalyst for organic synthesis . It has also been studied as a potential inhibitor of β-lactamase enzymes, specifically KPC-type carbapenemases .
Mode of Action
In the context of organic synthesis, 1,2-phenylenediboronic acid acts as a bidentate Lewis acid, catalyzing the inverse electron demand Diels–Alder reaction of 1,2-diazene . As a β-lactamase inhibitor, it forms a complex with the KPC-2 enzyme, enabling covalent binding to the catalytic Ser70 residue .
Biochemical Pathways
Its role as a catalyst in the inverse electron demand diels–alder reaction suggests it may influence pathways involving 1,2-diazene . As a β-lactamase inhibitor, it likely affects pathways related to bacterial resistance to β-lactam antibiotics .
Pharmacokinetics
Its potential as a β-lactamase inhibitor suggests it may have suitable bioavailability for therapeutic use .
Result of Action
As a catalyst, 1,2-phenylenediboronic acid facilitates the inverse electron demand Diels–Alder reaction of 1,2-diazene . As a β-lactamase inhibitor, it reduces the minimum inhibitory concentrations (MICs) of carbapenems, enhancing their effectiveness against KPC-producers .
Action Environment
The action of 1,2-phenylenediboronic acid can be influenced by environmental factors. For instance, its stability and ease of handling make it a more practical catalyst than some alternatives . Its effectiveness as a β-lactamase inhibitor may also be influenced by factors such as bacterial resistance mechanisms .
Orientations Futures
The glucose-sensitive self-adjusting drug delivery system simulates the physiological model of the human pancreas-secreting insulin and then precisely regulates the release of hypoglycemic drugs and controls the blood sugar . Therefore, it has good application prospects in the treatment of diabetes .
Propriétés
IUPAC Name |
(2-boronophenyl)boronic acid | |
---|---|---|
Source | PubChem | |
URL | https://pubchem.ncbi.nlm.nih.gov | |
Description | Data deposited in or computed by PubChem | |
InChI |
InChI=1S/C6H8B2O4/c9-7(10)5-3-1-2-4-6(5)8(11)12/h1-4,9-12H | |
Source | PubChem | |
URL | https://pubchem.ncbi.nlm.nih.gov | |
Description | Data deposited in or computed by PubChem | |
InChI Key |
ZKRVXVDQQOVOIM-UHFFFAOYSA-N | |
Source | PubChem | |
URL | https://pubchem.ncbi.nlm.nih.gov | |
Description | Data deposited in or computed by PubChem | |
Canonical SMILES |
B(C1=CC=CC=C1B(O)O)(O)O | |
Source | PubChem | |
URL | https://pubchem.ncbi.nlm.nih.gov | |
Description | Data deposited in or computed by PubChem | |
Molecular Formula |
C6H8B2O4 | |
Source | PubChem | |
URL | https://pubchem.ncbi.nlm.nih.gov | |
Description | Data deposited in or computed by PubChem | |
DSSTOX Substance ID |
DTXSID00623233 | |
Record name | 1,2-Phenylenediboronic acid | |
Source | EPA DSSTox | |
URL | https://comptox.epa.gov/dashboard/DTXSID00623233 | |
Description | DSSTox provides a high quality public chemistry resource for supporting improved predictive toxicology. | |
Molecular Weight |
165.75 g/mol | |
Source | PubChem | |
URL | https://pubchem.ncbi.nlm.nih.gov | |
Description | Data deposited in or computed by PubChem | |
Product Name |
1,2-Phenylenediboronic acid | |
CAS RN |
13506-83-7 | |
Record name | B,B′-1,2-Phenylenebis[boronic acid] | |
Source | CAS Common Chemistry | |
URL | https://commonchemistry.cas.org/detail?cas_rn=13506-83-7 | |
Description | CAS Common Chemistry is an open community resource for accessing chemical information. Nearly 500,000 chemical substances from CAS REGISTRY cover areas of community interest, including common and frequently regulated chemicals, and those relevant to high school and undergraduate chemistry classes. This chemical information, curated by our expert scientists, is provided in alignment with our mission as a division of the American Chemical Society. | |
Explanation | The data from CAS Common Chemistry is provided under a CC-BY-NC 4.0 license, unless otherwise stated. | |
Record name | 1,2-Phenylenediboronic acid | |
Source | EPA DSSTox | |
URL | https://comptox.epa.gov/dashboard/DTXSID00623233 | |
Description | DSSTox provides a high quality public chemistry resource for supporting improved predictive toxicology. | |
Q & A
Q1: How does the unique structure of 1,2-phenylenediboronic acid influence its interactions and applications?
A1: 1,2-Phenylenediboronic acid exhibits a propensity to form various supramolecular structures due to the presence of two boronic acid groups on the aromatic ring. [, , ] This feature facilitates the formation of cyclic semianhydrides, particularly in the presence of water or fluoride ions. [] Computational studies have shown that fluorination of the aromatic ring further stabilizes these cyclic structures. [] Furthermore, the molecule readily forms hydrogen-bonded networks, as exemplified by its ability to create nanotubular architectures capable of hosting water clusters. [] This self-assembling property makes it a promising candidate for applications in supramolecular chemistry and materials science, such as the design of hydrogen-bonded organic frameworks (HOFs). []
Q2: Can 1,2-phenylenediboronic acid be utilized in the development of luminescent materials?
A2: Yes, 1,2-phenylenediboronic acid can be complexed with appropriate ligands to create luminescent materials. Studies have shown its ability to form complexes with imidazo[1,2-a]pyridine derivatives, yielding blue-luminescent compounds. [] These complexes exhibit characteristic dimeric structures linked by the boronic acid groups, influencing their luminescent properties. [] The lack of significant charge transfer within these complexes, unlike some analogous systems, suggests that their emission properties are primarily governed by the chosen ligand. [] This finding highlights the potential of 1,2-phenylenediboronic acid as a building block for designing tailored luminescent materials by selecting suitable ligands.
Q3: How do substituents on the aromatic ring of 1,2-phenylenediboronic acid affect its reactivity?
A3: The reactivity of 1,2-phenylenediboronic acid can be significantly influenced by substituents on the aromatic ring. For instance, incorporating bromine atoms at the 2 and 6 positions facilitates a two-step synthesis of DATB (1,3-dioxa-5-aza-2,4,6-triborinane) derivatives. [] In this process, the brominated 1,2-phenylenediboronic acid undergoes Suzuki-Miyaura coupling followed by an acidic treatment to yield the desired DATB ring system. [] This example demonstrates how modifications to the parent 1,2-phenylenediboronic acid structure can be strategically employed to access other valuable heterocyclic compounds.
Avertissement et informations sur les produits de recherche in vitro
Veuillez noter que tous les articles et informations sur les produits présentés sur BenchChem sont destinés uniquement à des fins informatives. Les produits disponibles à l'achat sur BenchChem sont spécifiquement conçus pour des études in vitro, qui sont réalisées en dehors des organismes vivants. Les études in vitro, dérivées du terme latin "in verre", impliquent des expériences réalisées dans des environnements de laboratoire contrôlés à l'aide de cellules ou de tissus. Il est important de noter que ces produits ne sont pas classés comme médicaments et n'ont pas reçu l'approbation de la FDA pour la prévention, le traitement ou la guérison de toute condition médicale, affection ou maladie. Nous devons souligner que toute forme d'introduction corporelle de ces produits chez les humains ou les animaux est strictement interdite par la loi. Il est essentiel de respecter ces directives pour assurer la conformité aux normes légales et éthiques en matière de recherche et d'expérimentation.