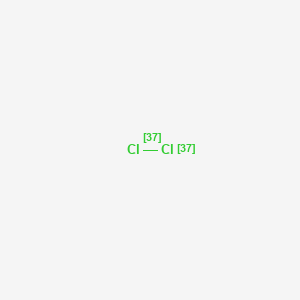
Chlorine, isotope of mass 37, at.
Vue d'ensemble
Description
Chlorine-37 is one of the stable isotopes of chlorine, the other being chlorine-35. Its nucleus contains 17 protons and 20 neutrons, giving it a total of 37 nucleons. Chlorine-37 accounts for approximately 24.23% of natural chlorine, with chlorine-35 making up the remaining 75.77% . This isotope has significant applications in various scientific fields, including chemistry, biology, and medicine.
Méthodes De Préparation
Synthetic Routes and Reaction Conditions
Chlorine-37 is not typically synthesized in a laboratory setting due to its natural abundance. it can be isolated from natural chlorine sources through isotopic separation techniques such as gas diffusion or centrifugation.
Industrial Production Methods
This process produces chlorine gas, sodium hydroxide, and hydrogen gas . The isotopic composition of chlorine produced industrially reflects the natural abundance of chlorine-37 and chlorine-35.
Analyse Des Réactions Chimiques
Types of Reactions
Chlorine-37, like other chlorine isotopes, undergoes various chemical reactions, including:
Oxidation: Chlorine can act as an oxidizing agent, reacting with metals to form metal chlorides.
Reduction: Chlorine can be reduced to chloride ions in reactions with reducing agents.
Substitution: Chlorine can participate in substitution reactions, particularly in organic chemistry, where it replaces hydrogen atoms in hydrocarbons.
Common Reagents and Conditions
Oxidation: Chlorine reacts with sodium to form sodium chloride.
Reduction: Chlorine can be reduced by hydrogen gas to form hydrochloric acid.
Substitution: Chlorine reacts with methane in the presence of ultraviolet light to form chloromethane and hydrogen chloride.
Major Products Formed
Oxidation: Metal chlorides (e.g., sodium chloride).
Reduction: Hydrochloric acid.
Substitution: Chlorinated hydrocarbons (e.g., chloromethane).
Applications De Recherche Scientifique
Chlorine-37 has several scientific research applications:
Neutrino Detection: Chlorine-37 is used in radiochemical methods for detecting solar neutrinos.
Isotopic Tracing: Chlorine-37 is used as an isotopic tracer in various chemical and environmental studies to track the movement and chemical reactions of chlorine in different systems.
Water Treatment: Chlorine, including chlorine-37, is widely used in water disinfection processes due to its strong oxidizing properties.
Mécanisme D'action
The mechanism of action of chlorine-37 in its applications is primarily based on its chemical reactivity:
Comparaison Avec Des Composés Similaires
Chlorine-37 can be compared with other chlorine isotopes, particularly chlorine-35:
Chlorine-35: This isotope has 17 protons and 18 neutrons, making it lighter than chlorine-37.
Uniqueness: The primary difference between chlorine-37 and chlorine-35 lies in their atomic mass and neutron count.
Similar Compounds
Chlorine-35: The most abundant isotope of chlorine.
Argon-37: A product of chlorine-37 transmutation in neutrino detection experiments.
Propriétés
Numéro CAS |
13981-73-2 |
---|---|
Formule moléculaire |
Cl2 |
Poids moléculaire |
73.931805 g/mol |
InChI |
InChI=1S/Cl2/c1-2/i1+2,2+2 |
Clé InChI |
KZBUYRJDOAKODT-XPULMUKRSA-N |
SMILES |
ClCl |
SMILES isomérique |
[37Cl][37Cl] |
SMILES canonique |
ClCl |
Origine du produit |
United States |
Synthesis routes and methods I
Procedure details
Synthesis routes and methods II
Procedure details
Synthesis routes and methods III
Procedure details
Synthesis routes and methods IV
Procedure details
Avertissement et informations sur les produits de recherche in vitro
Veuillez noter que tous les articles et informations sur les produits présentés sur BenchChem sont destinés uniquement à des fins informatives. Les produits disponibles à l'achat sur BenchChem sont spécifiquement conçus pour des études in vitro, qui sont réalisées en dehors des organismes vivants. Les études in vitro, dérivées du terme latin "in verre", impliquent des expériences réalisées dans des environnements de laboratoire contrôlés à l'aide de cellules ou de tissus. Il est important de noter que ces produits ne sont pas classés comme médicaments et n'ont pas reçu l'approbation de la FDA pour la prévention, le traitement ou la guérison de toute condition médicale, affection ou maladie. Nous devons souligner que toute forme d'introduction corporelle de ces produits chez les humains ou les animaux est strictement interdite par la loi. Il est essentiel de respecter ces directives pour assurer la conformité aux normes légales et éthiques en matière de recherche et d'expérimentation.