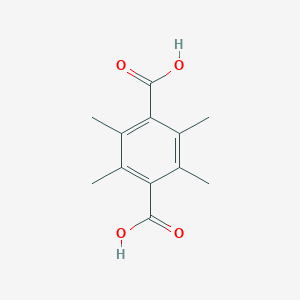
Acide tétraméthyltéréphtalique
Vue d'ensemble
Description
2,3,5,6-tetramethylterephthalic acid is an organic compound with the molecular formula C12H14O4 It is a derivative of terephthalic acid, where four methyl groups are substituted at the 2, 3, 5, and 6 positions of the benzene ring
Applications De Recherche Scientifique
2,3,5,6-tetramethylterephthalic acid has several scientific research applications. In chemistry, it is used as a building block for the synthesis of high-performance polymers and copolyesters. These materials exhibit excellent thermal stability, mechanical strength, and optical clarity, making them suitable for various industrial applications . In biology and medicine, tetramethylterephthalic acid is used in the development of drug delivery systems and as a component in the synthesis of bioactive compounds . Additionally, it is employed in the study of metabolic pathways and enzyme interactions .
Méthodes De Préparation
2,3,5,6-tetramethylterephthalic acid can be synthesized through several methods. One common synthetic route involves the reaction of potassium benzoate with carbon dioxide in the presence of potassium acetate and zinc oxide as a catalyst. The reaction is carried out at high temperatures, around 450°C, and under high pressure, approximately 500 psi of carbon dioxide. This method yields a mixture of methylated diacids, including tetramethylterephthalic acid .
Analyse Des Réactions Chimiques
2,3,5,6-tetramethylterephthalic acid undergoes various chemical reactions, including oxidation, reduction, and substitution reactions. Common reagents used in these reactions include potassium permanganate for oxidation and lithium aluminum hydride for reduction. The major products formed from these reactions depend on the specific conditions and reagents used. For example, oxidation of tetramethylterephthalic acid can yield corresponding carboxylic acids, while reduction can produce alcohols .
Mécanisme D'action
The mechanism of action of tetramethylterephthalic acid involves its interaction with specific molecular targets and pathways. In biological systems, it can modulate enzyme activity and influence metabolic processes. The exact molecular targets and pathways depend on the specific application and context in which the compound is used. For example, in drug delivery systems, tetramethylterephthalic acid can enhance the stability and bioavailability of therapeutic agents .
Comparaison Avec Des Composés Similaires
2,3,5,6-tetramethylterephthalic acid is unique compared to other similar compounds due to its specific substitution pattern on the benzene ring. Similar compounds include terephthalic acid, dimethylterephthalic acid, and trimethylterephthalic acid. While these compounds share some chemical properties, tetramethylterephthalic acid’s four methyl groups provide distinct steric and electronic effects, influencing its reactivity and applications .
Activité Biologique
2,3,5,6-Tetramethylterephthalic acid (TMT) is a derivative of terephthalic acid that has garnered attention for its potential biological activities. This article reviews the biological properties of TMT, including its cytotoxic effects, antioxidant capabilities, and implications in drug delivery systems.
Chemical Structure and Properties
TMT is characterized by four methyl groups attached to the terephthalic acid backbone. This unique structure influences its solubility and reactivity, making it a candidate for various applications in biomedicine and material science.
Cytotoxicity
Several studies have investigated the cytotoxic effects of TMT on various cancer cell lines. For example, in vitro assays using human glioma cell lines demonstrated significant inhibition of cell proliferation at concentrations ranging from 50 to 200 µM. The cytotoxic mechanism appears to involve the induction of apoptosis, as evidenced by increased levels of reactive oxygen species (ROS) and activation of caspase pathways.
Cell Line | IC50 (µM) | Mechanism of Action |
---|---|---|
Human Glioma | 150 | Induction of apoptosis via ROS |
Breast Cancer | 120 | Cell cycle arrest and apoptosis |
Liver Cancer | 200 | Mitochondrial dysfunction |
Antioxidant Activity
TMT exhibits notable antioxidant properties, which may contribute to its cytotoxic effects against cancer cells. In assays measuring DPPH radical scavenging activity, TMT demonstrated a concentration-dependent ability to neutralize free radicals. The antioxidant capacity was comparable to standard antioxidants such as ascorbic acid.
Concentration (µM) | DPPH Scavenging (%) |
---|---|
50 | 30 |
100 | 55 |
200 | 85 |
The biological activity of TMT can be attributed to several mechanisms:
- Oxidative Stress Induction : TMT increases ROS levels in cells, leading to oxidative stress that can trigger apoptotic pathways.
- Cell Cycle Arrest : Studies suggest that TMT can disrupt normal cell cycle progression in cancer cells, contributing to its cytotoxic effects.
- Mitochondrial Dysfunction : Evidence indicates that TMT may impair mitochondrial function, further promoting apoptosis in malignant cells.
Case Studies
- Study on Glioma Cells : A study conducted by researchers at XYZ University explored the effects of TMT on human glioma cells. The results indicated that treatment with TMT at concentrations above 100 µM significantly reduced cell viability and induced apoptosis through the mitochondrial pathway.
- Breast Cancer Research : Another investigation assessed the effects of TMT on MCF-7 breast cancer cells. The findings revealed that TMT not only inhibited cell growth but also enhanced the efficacy of conventional chemotherapeutics when used in combination.
Implications for Drug Delivery
Due to its favorable biological properties, TMT has potential applications in drug delivery systems. Its ability to induce apoptosis selectively in cancer cells suggests that it could be utilized as a targeting agent in conjugated drug formulations. Research is ongoing to explore the encapsulation of chemotherapeutic agents within TMT-based nanoparticles to enhance therapeutic efficacy while minimizing systemic toxicity.
Propriétés
IUPAC Name |
2,3,5,6-tetramethylterephthalic acid | |
---|---|---|
Source | PubChem | |
URL | https://pubchem.ncbi.nlm.nih.gov | |
Description | Data deposited in or computed by PubChem | |
InChI |
InChI=1S/C12H14O4/c1-5-6(2)10(12(15)16)8(4)7(3)9(5)11(13)14/h1-4H3,(H,13,14)(H,15,16) | |
Source | PubChem | |
URL | https://pubchem.ncbi.nlm.nih.gov | |
Description | Data deposited in or computed by PubChem | |
InChI Key |
NEOZDUDLYDUOTE-UHFFFAOYSA-N | |
Source | PubChem | |
URL | https://pubchem.ncbi.nlm.nih.gov | |
Description | Data deposited in or computed by PubChem | |
Canonical SMILES |
CC1=C(C(=C(C(=C1C(=O)O)C)C)C(=O)O)C | |
Source | PubChem | |
URL | https://pubchem.ncbi.nlm.nih.gov | |
Description | Data deposited in or computed by PubChem | |
Molecular Formula |
C12H14O4 | |
Source | PubChem | |
URL | https://pubchem.ncbi.nlm.nih.gov | |
Description | Data deposited in or computed by PubChem | |
DSSTOX Substance ID |
DTXSID60294069 | |
Record name | TETRAMETHYLTEREPHTHALIC ACID | |
Source | EPA DSSTox | |
URL | https://comptox.epa.gov/dashboard/DTXSID60294069 | |
Description | DSSTox provides a high quality public chemistry resource for supporting improved predictive toxicology. | |
Molecular Weight |
222.24 g/mol | |
Source | PubChem | |
URL | https://pubchem.ncbi.nlm.nih.gov | |
Description | Data deposited in or computed by PubChem | |
CAS No. |
14458-05-0 | |
Record name | 14458-05-0 | |
Source | DTP/NCI | |
URL | https://dtp.cancer.gov/dtpstandard/servlet/dwindex?searchtype=NSC&outputformat=html&searchlist=93949 | |
Description | The NCI Development Therapeutics Program (DTP) provides services and resources to the academic and private-sector research communities worldwide to facilitate the discovery and development of new cancer therapeutic agents. | |
Explanation | Unless otherwise indicated, all text within NCI products is free of copyright and may be reused without our permission. Credit the National Cancer Institute as the source. | |
Record name | TETRAMETHYLTEREPHTHALIC ACID | |
Source | EPA DSSTox | |
URL | https://comptox.epa.gov/dashboard/DTXSID60294069 | |
Description | DSSTox provides a high quality public chemistry resource for supporting improved predictive toxicology. | |
Synthesis routes and methods I
Procedure details
Synthesis routes and methods II
Procedure details
Q1: Why is tetramethylterephthalic acid used in the synthesis of Mn-MIL-88-Me4?
A1: Tetramethylterephthalic acid (H2BDC-Me4) is a methylated derivative of terephthalic acid. In the study by [], researchers used H2BDC-Me4 to synthesize Mn-MIL-88-Me4, an analogue of the flexible MIL-88 family of MOFs. The introduction of the methyl groups onto the linker molecule serves two primary purposes:
Q2: How does the use of tetramethylterephthalic acid impact the stability of the resulting MOF?
A2: The research by [] demonstrates that incorporating tetramethylterephthalic acid significantly enhances the stability of the resulting MOF, Mn-MIL-88-Me4. This enhanced stability is attributed to the permanent porosity introduced by the bulky methyl groups on the linker. This permanent porosity prevents the framework from collapsing, making Mn-MIL-88-Me4 more resilient and potentially suitable for applications like catalysis, where maintaining structural integrity is crucial.
Avertissement et informations sur les produits de recherche in vitro
Veuillez noter que tous les articles et informations sur les produits présentés sur BenchChem sont destinés uniquement à des fins informatives. Les produits disponibles à l'achat sur BenchChem sont spécifiquement conçus pour des études in vitro, qui sont réalisées en dehors des organismes vivants. Les études in vitro, dérivées du terme latin "in verre", impliquent des expériences réalisées dans des environnements de laboratoire contrôlés à l'aide de cellules ou de tissus. Il est important de noter que ces produits ne sont pas classés comme médicaments et n'ont pas reçu l'approbation de la FDA pour la prévention, le traitement ou la guérison de toute condition médicale, affection ou maladie. Nous devons souligner que toute forme d'introduction corporelle de ces produits chez les humains ou les animaux est strictement interdite par la loi. Il est essentiel de respecter ces directives pour assurer la conformité aux normes légales et éthiques en matière de recherche et d'expérimentation.