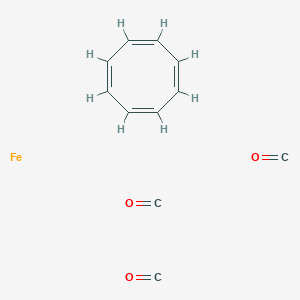
Cyclooctatetraène Tricarbonyle de Fer
- Cliquez sur DEMANDE RAPIDE pour recevoir un devis de notre équipe d'experts.
- Avec des produits de qualité à un prix COMPÉTITIF, vous pouvez vous concentrer davantage sur votre recherche.
Vue d'ensemble
Description
Tricarbonyl(cyclooctatetraene)iron is an organoiron compound with the formula (C₈H₈)Fe(CO)₃. It is an orange, diamagnetic solid and is known for its unique structure where the iron center is coordinated to a cyclooctatetraene ligand and three carbonyl groups . This compound is a member of the (diene)Fe(CO)₃ complexes and is notable for its stability and reactivity in various chemical processes .
Applications De Recherche Scientifique
Tricarbonyl(cyclooctatetraene)iron has a wide range of applications in scientific research:
Safety and Hazards
Tricarbonyl(cyclooctatetraene)iron(II) is toxic if swallowed, in contact with skin, or if inhaled . It is recommended to avoid dust formation, breathing mist, gas, or vapors, and contact with skin and eyes . Use of personal protective equipment, chemical impermeable gloves, and ensuring adequate ventilation is advised .
Orientations Futures
Mécanisme D'action
Target of Action
Cyclooctatetraene Iron Tricarbonyl, also known as (Cyclooctatetraene)iron tricarbonyl or Tricarbonyl(cyclooctatetraene)iron(II), is an organoiron compound
Action Environment
The action, efficacy, and stability of Cyclooctatetraene Iron Tricarbonyl are likely to be influenced by a variety of environmental factors. These could include temperature, pH, the presence of other reactants, and the specific physical and chemical properties of the environment. For example, the compound is known to be an orange, diamagnetic solid , suggesting that it could be sensitive to changes in temperature or pressure.
Méthodes De Préparation
Synthetic Routes and Reaction Conditions
Tricarbonyl(cyclooctatetraene)iron can be synthesized through the reaction of cyclooctatetraene with iron pentacarbonyl. The reaction typically involves heating the reactants under reflux conditions in an inert atmosphere to prevent oxidation . The general reaction is as follows:
C8H8+Fe(CO)5→(C8H8)Fe(CO)3+2CO
The product is then purified through recrystallization or sublimation to obtain the pure compound .
Industrial Production Methods
While specific industrial production methods for Tricarbonyl(cyclooctatetraene)iron are not widely documented, the synthesis generally follows similar principles as laboratory methods, with adjustments for scale and efficiency. Industrial processes would likely involve continuous flow reactors and automated systems to ensure consistent quality and yield .
Analyse Des Réactions Chimiques
Types of Reactions
Tricarbonyl(cyclooctatetraene)iron undergoes various types of chemical reactions, including:
Oxidation: The compound can be oxidized to form different iron oxidation states.
Reduction: It can be reduced to form iron(0) complexes.
Substitution: The carbonyl ligands can be substituted with other ligands such as phosphines or amines.
Common Reagents and Conditions
Common reagents used in these reactions include oxidizing agents like hydrogen peroxide for oxidation, reducing agents like sodium borohydride for reduction, and various nucleophiles for substitution reactions . The reactions are typically carried out under controlled temperatures and inert atmospheres to prevent unwanted side reactions .
Major Products
The major products formed from these reactions depend on the specific conditions and reagents used. For example, substitution reactions can yield a variety of iron complexes with different ligands, while oxidation and reduction reactions can produce iron(III) or iron(0) species .
Comparaison Avec Des Composés Similaires
Similar Compounds
Cyclopentadienyl iron dicarbonyl dimer: Another organoiron compound with similar catalytic properties.
Iron pentacarbonyl: A precursor used in the synthesis of Tricarbonyl(cyclooctatetraene)iron and other iron carbonyl complexes.
Diironnonacarbonyl: A related iron carbonyl complex with different reactivity and applications.
Uniqueness
Tricarbonyl(cyclooctatetraene)iron is unique due to its stable coordination with the cyclooctatetraene ligand, which imparts distinct reactivity and stability compared to other iron carbonyl complexes. Its ability to undergo various chemical transformations and its applications in diverse fields make it a valuable compound in both research and industry .
Propriétés
{ "Design of the Synthesis Pathway": "The synthesis of Tricarbonyl(cyclooctatetraene)iron(II) can be achieved through the reaction of cyclooctatetraene with iron pentacarbonyl in the presence of a reducing agent such as sodium amalgam.", "Starting Materials": [ "Cyclooctatetraene", "Iron pentacarbonyl", "Sodium amalgam" ], "Reaction": [ "Add cyclooctatetraene to a flask containing iron pentacarbonyl.", "Add sodium amalgam to the flask to act as a reducing agent.", "Heat the mixture under reflux for several hours.", "Cool the mixture and filter the resulting solid.", "Wash the solid with diethyl ether to remove any impurities.", "Dry the solid under vacuum to obtain Tricarbonyl(cyclooctatetraene)iron(II)." ] } | |
Numéro CAS |
12093-05-9 |
Formule moléculaire |
C11H14FeO3 |
Poids moléculaire |
250.07 g/mol |
Nom IUPAC |
cyclooctatetraene;formaldehyde;iron |
InChI |
InChI=1S/C8H8.3CH2O.Fe/c1-2-4-6-8-7-5-3-1;3*1-2;/h1-8H;3*1H2;/b2-1-,3-1?,4-2?,5-3-,6-4-,7-5?,8-6?,8-7-;;;; |
Clé InChI |
VKMWLPMIRGHDAA-JGZYGLCTSA-N |
SMILES isomérique |
C=O.C=O.C=O.C\1=C\C=C/C=C\C=C1.[Fe] |
SMILES |
C=O.C=O.C=O.C1=CC=CC=CC=C1.[Fe] |
SMILES canonique |
C=O.C=O.C=O.C1=CC=CC=CC=C1.[Fe] |
Pictogrammes |
Irritant |
Origine du produit |
United States |
Avertissement et informations sur les produits de recherche in vitro
Veuillez noter que tous les articles et informations sur les produits présentés sur BenchChem sont destinés uniquement à des fins informatives. Les produits disponibles à l'achat sur BenchChem sont spécifiquement conçus pour des études in vitro, qui sont réalisées en dehors des organismes vivants. Les études in vitro, dérivées du terme latin "in verre", impliquent des expériences réalisées dans des environnements de laboratoire contrôlés à l'aide de cellules ou de tissus. Il est important de noter que ces produits ne sont pas classés comme médicaments et n'ont pas reçu l'approbation de la FDA pour la prévention, le traitement ou la guérison de toute condition médicale, affection ou maladie. Nous devons souligner que toute forme d'introduction corporelle de ces produits chez les humains ou les animaux est strictement interdite par la loi. Il est essentiel de respecter ces directives pour assurer la conformité aux normes légales et éthiques en matière de recherche et d'expérimentation.