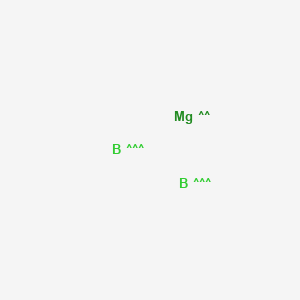
Magnesium boride
Vue d'ensemble
Description
Magnesium boride (MgB₂) is an intermetallic compound with a hexagonal crystal structure, composed of alternating layers of magnesium and boron. The boron layers adopt a honeycomb lattice similar to graphite, while magnesium atoms occupy interstitial sites . Discovered as a superconductor in 2001, MgB₂ exhibits a critical temperature (Tₐ) of 39 K, the highest among simple binary compounds . Its superconducting properties arise from multiband electronic interactions, where two distinct energy gaps (s- and p-bands) contribute to Cooper pair formation . Beyond superconductivity, MgB₂ is used in high-energy fuels due to its combustion efficiency and in ceramic composites for enhanced mechanical properties .
Méthodes De Préparation
Solid-State Synthesis Methods
Solid-state synthesis remains the most widely used technique for magnesium boride production due to its scalability and straightforward implementation. This method involves direct reaction between magnesium and boron precursors under controlled atmospheres and temperatures.
Conventional Solid-State Reaction
The conventional approach employs stoichiometric mixtures of magnesium metal and amorphous boron powder. As detailed in U.S. Patent 6,511,943 , optimal results are achieved with a Mg:B molar ratio exceeding 0.6:1, typically 1:1, to compensate for magnesium volatility at high temperatures. The reactants are sealed in tantalum or molybdenum foil to prevent oxidation and heated under inert argon gas at 700–900°C for 1–2 hours. This method yields MgB₂ with a critical transition temperature () of 39 K, though residual unreacted Mg or B impurities may persist if stoichiometry deviates .
A critical advancement involves using boron pellets (1–10 mm diameter) pressed from fine powder (325 mesh), which enhances surface area and reaction kinetics. Post-synthesis, the product is washed with distilled water to remove excess MgO, followed by drying at 70–80°C . X-ray diffraction (XRD) analysis confirms predominant MgB₂ formation with minor MgO peaks, attributable to atmospheric exposure during handling .
Modified Solid-State Approaches
Recent modifications focus on mitigating magnesium loss. For instance, mechanical pre-activation of reactants via planetary ball milling (400 rpm, 4–10 hours) under argon reduces the required synthesis temperature to 650–700°C . This pre-treatment fractures boron aggregates, increasing reactive surfaces and enabling lower-energy diffusion pathways. Post-milling, the mixture is heated under 0.5–1 atm hydrogen backpressure, which suppresses magnesium sublimation and promotes boride nucleation . The resultant MgB₂ exhibits narrower superconducting transitions ( K) compared to non-milled samples, as hydrogen backfilling minimizes oxygen contamination .
Mechanical Alloying and Ball Milling
Mechanical alloying leverages high-energy deformation to induce chemical reactions at reduced temperatures, bypassing the limitations of conventional solid-state methods.
High-Energy Ball Milling
In ex-situ powder-in-tube (PIT) processing, pre-synthesized MgB₂ powders are ball-milled to refine grain size and enhance current-carrying capacity. A study by Applied Superconductivity demonstrated that milling MgB₂ at 180–240 rpm for 72–144 hours reduces average grain size from 1 μm to 50 nm, elevating critical current density () to A/cm² at 4 T and 5 K. Prolonged milling (>200 hours), however, introduces lattice strain and amorphous regions, degrading superconducting coherence .
Low-Temperature Synthesis via Mechanical Activation
Combining milling with low-temperature annealing offers a hybrid route. For example, milling MgCl₂, Ni, and NaBH₄ at 400 rpm for 4 hours under argon enables subsequent reaction at 700°C to form ternary MgNi₂.₅B₂ . The borohydride precursor decomposes during heating, releasing atomic boron that reacts with Mg and Ni to yield a multi-phase product (MgB₂, Ni₂B). This method achieves 95% phase purity, with impurity fractions controlled by adjusting milling duration and boron stoichiometry .
Chemical Vapor Deposition (CVD)
CVD is uniquely suited for depositing this compound thin films, critical for electronic and coating applications.
Precursor Selection and Deposition Parameters
Crociani et al. synthesized MgB₂ films using Mg(BH₄)₂ as a single-source precursor. Deposition at 400–500°C under 10⁻² mbar argon yields amorphous films, which crystallize upon post-annealing at 600°C. XPS depth profiling reveals a composition gradient, with boron-rich layers (B:Mg ≈ 2.3:1) at the substrate interface and stoichiometric MgB₂ at the surface . Film adhesion is enhanced by using nickel or stainless steel substrates, though interfacial MgO formation remains a challenge .
Thermodynamic Considerations and Phase Stability
The Mg-B phase diagram, reconstructed via CALPHAD modeling , provides critical insights into synthesis conditions required for phase-pure MgB₂.
Mg-B Phase Diagram Analysis
At atmospheric pressure, MgB₂ decomposes peritectically at 1550°C into MgB₄ and gaseous Mg . However, under hydrogen backpressure (0.5–1 atm), the decomposition temperature shifts to 1750°C, stabilizing MgB₂ during high-temperature synthesis . The diagram also predicts that MgB₇ forms above 2150°C, though kinetic barriers often prevent its formation in standard reactors .
Influence of Synthesis Temperature and Pressure
Thermodynamic simulations reveal that Mg loss—a major hurdle in conventional methods—is minimized at pressures >5 atm. For instance, sintering at 920°C under 5 atm argon increases MgB₂ yield from 78% to 94% by suppressing Mg sublimation . Similarly, hydrogen’s reducing action mitigates oxide contamination, as evidenced by oxygen content <2 at.% in CVD films deposited under H₂/Ar mixtures .
Comparative Analysis of Synthesis Methods
The table below summarizes key parameters and outcomes across methods:
Analyse Des Réactions Chimiques
Solid-State Reaction
The simplest method involves heating magnesium and boron powders above 650°C under inert conditions:
-
Conditions : Temperatures ≥650°C, inert atmosphere (Ar/N₂) .
-
Mechanism : Magnesium vapor diffuses into boron grain boundaries due to its low melting point (652°C) .
Hydrolysis Reactions
MgB₂ reacts with water and acids, producing hydrogen and boron-containing compounds:
With Water
-
Notable : No boranes (e.g., B₂H₆) or boric acid (H₃BO₃) are formed, contrary to initial expectations .
With Dilute Acids
Reaction with HCl or H₂SO₄ releases hydrogen and trace boranes:
Combustion and Pyrolytic Reactions
MgB₂ burns completely in oxygen or oxidizing environments:
-
Applications : Used in decoy flares with 30–60% higher spectral efficiency than traditional magnesium flares .
Reactions with Hydroxides
MgB₂ reacts with KOH to form borohydrides:
Intermetallic Compound Formation
MgB₂ participates in reactions with transition metals to form ternary borides:
With Nickel
At 670–960°C, MgB₂ reacts with Ni to form MgNi₃B₂:
-
Conditions : Autogenous pressure (>1.7 MPa) and NaBH₄ as a boron source .
-
Magnetic Properties : Ferromagnetic behavior observed in core-shell aggregates .
Thermodynamic Stability and Decomposition
MgB₂ decomposes at high temperatures under varying pressures:
Compound | Decomposition Temperature (°C) | Enthalpy of Formation (kJ/mol·atoms) |
---|---|---|
MgB₂ | 1,545 | -18.55 ± 1.12 |
MgB₄ | 1,735 | -14.73 ± 0.50 |
MgB₇ | 2,150 | -13.39 ± 0.30 |
Reactions with Boron Oxide
MgB₂ can form via reduction of B₂O₃ in the presence of excess Mg:
Superconductivity-Related Reactions
MgB₂’s superconducting properties (Tₐ = 39 K) are retained in wire forms produced via:
Table 1: Reaction Conditions and Products
Table 2: Thermodynamic Data for Mg-B System
Reaction | ΔG (kJ/mol) | Temperature (°C) |
---|---|---|
Mg (l) + 2B (s) → MgB₂ (s) | -86 | 500 |
3Mg (l) + B₂O₃ (l) → 3MgO + 2B | -488 | 500 |
4Mg + B₂O₃ → MgB₂ + 3MgO | -569 | 500 |
Applications De Recherche Scientifique
Superconductivity
Overview
Magnesium boride is recognized as a low-temperature superconductor with a critical temperature () of approximately 39 K. Its superconducting properties make it suitable for various applications in electronics and power systems.
Applications
- Superconducting Wires and Cables : MgB₂ wires are being developed for use in high-efficiency power transmission systems. The ability to carry high currents without resistance can significantly reduce energy losses in electrical grids .
- Magnetic Resonance Imaging (MRI) : Due to its superconducting properties, MgB₂ is considered for use in MRI machines, offering potential benefits such as reduced operational costs and improved imaging quality .
- Cryogen-Free Magnets : The compound's efficiency at higher temperatures compared to traditional superconductors allows for the development of cryogen-free magnetic systems, which are more practical and cost-effective .
Energy Storage
Overview
MgB₂ has been investigated for its potential in energy storage applications due to its lightweight and high-energy density characteristics.
Applications
- Hydrogen Storage : Research indicates that this compound can be utilized as a hydrogen storage material. Its ability to absorb hydrogen at relatively low temperatures makes it a candidate for clean energy technologies .
- High-Energy Fuels : this compound has been explored as a high-energy fuel alternative. Studies show that sintered MgB₂ exhibits favorable combustion properties, making it suitable for various energetic applications .
Materials Science
Overview
The unique structural properties of this compound lend themselves to advancements in materials science.
Applications
- Thin Films and Coatings : this compound films can be synthesized using chemical vapor deposition (CVD) techniques. These films have potential applications in electronic devices and protective coatings due to their hardness and thermal stability .
- Composite Materials : The incorporation of MgB₂ into composite materials can enhance mechanical properties, making them suitable for aerospace and automotive applications where lightweight and strength are critical .
Environmental Remediation
Overview
The environmental applications of this compound focus on its potential as a sorbent material.
Applications
- Pollutant Removal : Research indicates that this compound can effectively capture pollutants from industrial emissions. Its reactive properties allow it to bind with harmful substances, thereby reducing environmental impact .
- Waste Treatment : MgB₂ has been studied for its ability to treat wastewater by adsorbing heavy metals and other contaminants, showcasing its versatility in environmental management .
Summary Table of Applications
Application Area | Specific Use Case | Benefits |
---|---|---|
Superconductivity | Wires and cables for power transmission | Reduced energy loss, high current capacity |
MRI machines | Cost-effective operation | |
Cryogen-free magnets | Practicality in various settings | |
Energy Storage | Hydrogen storage | Low temperature absorption |
High-energy fuels | Favorable combustion properties | |
Materials Science | Thin films and coatings | Hardness, thermal stability |
Composite materials | Enhanced mechanical properties | |
Environmental Remediation | Pollutant removal | Reduces harmful emissions |
Wastewater treatment | Adsorption of heavy metals |
Mécanisme D'action
The superconducting mechanism of magnesium diboride is primarily described by BCS theory. It involves the formation of Cooper pairs of electrons that move through the lattice without resistance. Magnesium boride has two types of electrons at the Fermi level with different behaviors: sigma-bonding electrons, which are strongly superconducting, and pi-bonding electrons, which are less so . This dual-band superconductivity is unique and contributes to its high critical temperature.
Comparaison Avec Des Composés Similaires
Structural and Compositional Similarities
Aluminum Diboride (AlB₂)
- Structure : AlB₂ shares MgB₂’s hexagonal layered structure, with boron in a graphite-like arrangement and aluminum in interlayer sites. Both compounds belong to the AlB₂ structure family .
- Electrical Conductivity: AlB₂ exhibits metallic conductivity along the hexagonal plane, akin to MgB₂.
- Reactivity : AlB₂ reacts with acids to release toxic borane gas, limiting its industrial use compared to MgB₂ .
Cobalt Borides (CoB, Co₂B)
- Structure : CoB and Co₂B form orthorhombic and tetragonal phases, respectively, distinct from MgB₂’s hexagonal symmetry. Boriding of Co–Mg alloys produces surface layers with CoB and Co₂B phases, enhancing hardness .
- Mechanical Properties : Borided Co–Mg alloys achieve hardness values of 1,674–1,956 HV₀.₀₅, far exceeding untreated cobalt (52 HV₀.₀₅) .
Higher Magnesium Borides (MgB₄, MgB₇, MgB₁₂)
- Structure : MgB₄ and MgB₇ exhibit complex boron-rich frameworks. MgB₇ has a higher boron content, leading to stronger shear resistance under pressure .
Elemental Boron
- Combustion Performance : While boron has high combustion enthalpy, its surface oxide layer impedes ignition. MgB₂ overcomes this via magnesium evaporation, which disrupts oxide formation, achieving 20% higher combustion efficiency .
Mechanical and Thermal Properties
Hardness and Wear Resistance
MgB₂’s nano-hardness is overshadowed by higher borides like MgB₇, which resists shear deformation up to 40 GPa .
Thermal Stability
- Combustion : MgB₂ releases 45.2 kJ/g of energy, outperforming amorphous boron (37.5 kJ/g) .
- Debye Temperature : MgB₂ (ΘD = 750 K) has lower thermal expansion than MgB₇ (ΘD = 1,050 K), reflecting stronger atomic bonding in boron-rich phases .
Electrical and Superconducting Properties
MgB₂’s superconductivity is attributed to strong electron-phonon coupling in its s-band, while the p-band remains metallic . Doping with carbon or titanium improves its magnetic field tolerance .
Activité Biologique
Magnesium boride (MgB₂) is a compound that has garnered attention for its potential biological activities, particularly in the fields of medicine and biotechnology. This article delves into the biological properties of this compound, highlighting its antioxidant, antimicrobial, and wound healing capabilities based on recent research findings.
Overview of this compound
This compound is a binary compound consisting of magnesium and boron. It is primarily known for its superconducting properties but has recently been investigated for various biological applications. The unique structural characteristics of this compound, particularly in nanosheet form, enhance its interaction with biological systems.
1. Antioxidant Activity
Recent studies have shown that MgB₂ nanosheets exhibit significant antioxidant properties. For instance, a study reported that these nanosheets demonstrated an antioxidant activity of 75.24 ± 4.15% at a concentration of 200 mg/L . This property is crucial as antioxidants play a vital role in neutralizing free radicals, thereby preventing oxidative stress in cells .
2. Antimicrobial Effects
This compound has been evaluated for its antimicrobial properties against various bacterial strains. In one study, MgB₂ nanosheets were shown to completely inhibit microbial cell viability at concentrations as low as 12.5 mg/L , with cell viability inhibition reaching 99.7 ± 5.78% at this concentration . The compound's mechanism involves disrupting bacterial membranes through the release of magnesium ions, which enhances its bactericidal effects.
3. Wound Healing Properties
The application of this compound in wound healing has been explored through a novel approach involving reactive metal boride nanoparticles (MB NPs). These nanoparticles can hydrolyze to produce a local alkaline environment and generate boron dihydroxy groups that trap lipopolysaccharides (LPS) released from dead bacteria. This dual action not only enhances bacterial death but also mitigates inflammation caused by LPS, significantly promoting wound healing .
Case Study 1: Antioxidant and Antimicrobial Evaluation
A detailed evaluation was conducted on MgB₂ nanosheets where their antioxidant and antimicrobial activities were assessed:
Concentration (mg/L) | Antioxidant Activity (%) | Cell Viability Inhibition (%) |
---|---|---|
12.5 | - | 99.7 ± 5.78 |
25 | - | 99.89 ± 6.02 |
50 | - | 100 ± 5.84 |
200 | 75.24 ± 4.15 | - |
This data illustrates the potent biological activity of MgB₂ at varying concentrations, emphasizing its potential as an antimicrobial agent .
Case Study 2: Wound Healing Mechanism
In vivo studies demonstrated the effectiveness of this compound nanoparticles in accelerating wound healing in infected models:
- Mechanism : Hydrolysis of MB NPs generates an alkaline microenvironment that traps inflammatory mediators while promoting bacterial death.
- Outcome : Enhanced healing rates were observed in infected wounds treated with MB NPs compared to controls, showcasing the compound's therapeutic potential .
Research Findings and Implications
Research indicates that this compound's unique properties can be harnessed for various biomedical applications:
- Biocompatibility : Studies suggest that MgB₂ exhibits favorable biocompatibility, making it suitable for applications in drug delivery systems and tissue engineering.
- Hydrogen Storage : While not directly related to biological activity, the ability of modified magnesium borides to store hydrogen efficiently may have implications for developing bioenergetic systems .
Q & A
Q. What are the primary synthesis methods for magnesium boride (MgB₂) and their respective advantages in laboratory settings?
Basic Research Focus
MgB₂ is synthesized via:
- Solid-state reaction : Mixing stoichiometric Mg and B powders under inert atmosphere, followed by high-temperature sintering (~800–1000°C) .
- Aluminum/Magnesium Thermal Reduction : Reducing boron oxides with Mg or Al at elevated temperatures (e.g., ) .
- Sintering Alloys : Combining Mg, Al, and B via high-temperature sintering to create ternary alloys (e.g., Mg₀.₅Al₀.₅B₂) for enhanced combustion properties .
Methodological Considerations :
- Optimize sintering time (e.g., 2–4 hours) and temperature (700–900°C) to minimize impurities .
- Use XRD and EDS to validate phase purity and elemental distribution .
Q. How do the structural properties of MgB₂ influence its superconducting behavior?
Basic Research Focus
MgB₂ exhibits a hexagonal lattice with alternating Mg and B layers. The B layers form a honeycomb structure analogous to graphite, enabling strong electron-phonon coupling. This contributes to its multi-band superconductivity (two σ-bands from B and one π-band from Mg), with a critical temperature () of ~39 K .
Key Insights :
- σ-bands dominate superconductivity, while π-bands contribute to electrical conductivity .
- Structural defects (e.g., grain boundaries) can suppress , necessitating high-purity synthesis .
Q. What experimental approaches are used to enhance the superconducting transition temperature (TcT_cTc) of MgB₂?
Advanced Research Focus
- Electron Doping : Substituting Mg with atoms like C or Al (e.g., Mg₁₋ₓAlₓB₂) increases by altering carrier density .
- Biaxial Strain : Applying tensile strain (e.g., via substrate mismatch) enhances by modifying interatomic distances .
- 2D Heterostructures : Combining MgB₂ with graphene improves thermal stability and retention at higher magnetic fields .
Methodological Tools :
- Density functional theory (DFT) simulations to predict dopant effects .
- Transport measurements (e.g., resistivity, Hall effect) to quantify shifts .
Q. In combustion studies, how does MgB₂ compare to amorphous boron in terms of efficiency?
Advanced Research Focus
MgB₂ outperforms boron due to:
- Higher Combustion Heat : MgB₂ releases ~58 kJ/g vs. boron’s ~43 kJ/g in oxygenated environments .
- Suppressed Oxide Layer Formation : Mg evaporation during combustion disrupts surface oxide layers, enhancing oxidation efficiency .
Parameter | MgB₂ | Amorphous Boron |
---|---|---|
Combustion Heat (kJ/g) | 58 | 43 |
Efficiency (%) | 92 | 78 |
Key Analysis Method | Oxygen bomb calorimetry, EDS |
Q. What challenges arise in synthesizing 2D MgB₂ structures, and how are they characterized?
Advanced Research Focus
- Synthesis Challenges : Achieving monolayer stability and preventing layer aggregation during exfoliation .
- Characterization Techniques :
Recent Advances :
- Strain engineering via substrate interaction improves in 2D MgB₂/graphene hybrids .
Q. How does multi-band superconductivity in MgB₂ complicate transport measurements?
Advanced Research Focus
- Band-Specific Contributions : σ-bands (high ) and π-bands (lower ) create overlapping superconducting gaps .
- Resolution Strategies :
Q. What methodological considerations are critical for evaluating MgB₂ in hydrogen storage?
Advanced Research Focus
- Cycling Kinetics : Modify MgB₂ with catalysts (e.g., Ti, Fe) to accelerate adsorption/desorption .
- Capacity Targets : Aim for >5 wt% capacity via nanostructuring (e.g., porous MgB₂) .
- Analysis Tools :
- Sieverts apparatus for uptake measurements.
- TGA-DSC to study decomposition pathways .
Q. What safety protocols are recommended for high-temperature MgB₂ experiments?
Basic Research Focus
Propriétés
InChI |
InChI=1S/2B.Mg | |
---|---|---|
Source | PubChem | |
URL | https://pubchem.ncbi.nlm.nih.gov | |
Description | Data deposited in or computed by PubChem | |
InChI Key |
PZKRHHZKOQZHIO-UHFFFAOYSA-N | |
Source | PubChem | |
URL | https://pubchem.ncbi.nlm.nih.gov | |
Description | Data deposited in or computed by PubChem | |
Canonical SMILES |
[B].[B].[Mg] | |
Source | PubChem | |
URL | https://pubchem.ncbi.nlm.nih.gov | |
Description | Data deposited in or computed by PubChem | |
Molecular Formula |
B2Mg | |
Record name | magnesium boride | |
Source | Wikipedia | |
URL | https://en.wikipedia.org/wiki/Magnesium_boride | |
Description | Chemical information link to Wikipedia. | |
Source | PubChem | |
URL | https://pubchem.ncbi.nlm.nih.gov | |
Description | Data deposited in or computed by PubChem | |
Molecular Weight |
45.93 g/mol | |
Source | PubChem | |
URL | https://pubchem.ncbi.nlm.nih.gov | |
Description | Data deposited in or computed by PubChem | |
Physical Description |
Grey powder; [Sigma-Aldrich MSDS] | |
Record name | Magnesium diboride | |
Source | Haz-Map, Information on Hazardous Chemicals and Occupational Diseases | |
URL | https://haz-map.com/Agents/15312 | |
Description | Haz-Map® is an occupational health database designed for health and safety professionals and for consumers seeking information about the adverse effects of workplace exposures to chemical and biological agents. | |
Explanation | Copyright (c) 2022 Haz-Map(R). All rights reserved. Unless otherwise indicated, all materials from Haz-Map are copyrighted by Haz-Map(R). No part of these materials, either text or image may be used for any purpose other than for personal use. Therefore, reproduction, modification, storage in a retrieval system or retransmission, in any form or by any means, electronic, mechanical or otherwise, for reasons other than personal use, is strictly prohibited without prior written permission. | |
CAS No. |
12007-25-9 | |
Record name | Magnesium boride (MgB2) | |
Source | CAS Common Chemistry | |
URL | https://commonchemistry.cas.org/detail?cas_rn=12007-25-9 | |
Description | CAS Common Chemistry is an open community resource for accessing chemical information. Nearly 500,000 chemical substances from CAS REGISTRY cover areas of community interest, including common and frequently regulated chemicals, and those relevant to high school and undergraduate chemistry classes. This chemical information, curated by our expert scientists, is provided in alignment with our mission as a division of the American Chemical Society. | |
Explanation | The data from CAS Common Chemistry is provided under a CC-BY-NC 4.0 license, unless otherwise stated. | |
Record name | Magnesium boride (MgB2) | |
Source | EPA Chemicals under the TSCA | |
URL | https://www.epa.gov/chemicals-under-tsca | |
Description | EPA Chemicals under the Toxic Substances Control Act (TSCA) collection contains information on chemicals and their regulations under TSCA, including non-confidential content from the TSCA Chemical Substance Inventory and Chemical Data Reporting. | |
Record name | Magnesium diboride | |
Source | European Chemicals Agency (ECHA) | |
URL | https://echa.europa.eu/substance-information/-/substanceinfo/100.031.352 | |
Description | The European Chemicals Agency (ECHA) is an agency of the European Union which is the driving force among regulatory authorities in implementing the EU's groundbreaking chemicals legislation for the benefit of human health and the environment as well as for innovation and competitiveness. | |
Explanation | Use of the information, documents and data from the ECHA website is subject to the terms and conditions of this Legal Notice, and subject to other binding limitations provided for under applicable law, the information, documents and data made available on the ECHA website may be reproduced, distributed and/or used, totally or in part, for non-commercial purposes provided that ECHA is acknowledged as the source: "Source: European Chemicals Agency, http://echa.europa.eu/". Such acknowledgement must be included in each copy of the material. ECHA permits and encourages organisations and individuals to create links to the ECHA website under the following cumulative conditions: Links can only be made to webpages that provide a link to the Legal Notice page. | |
Avertissement et informations sur les produits de recherche in vitro
Veuillez noter que tous les articles et informations sur les produits présentés sur BenchChem sont destinés uniquement à des fins informatives. Les produits disponibles à l'achat sur BenchChem sont spécifiquement conçus pour des études in vitro, qui sont réalisées en dehors des organismes vivants. Les études in vitro, dérivées du terme latin "in verre", impliquent des expériences réalisées dans des environnements de laboratoire contrôlés à l'aide de cellules ou de tissus. Il est important de noter que ces produits ne sont pas classés comme médicaments et n'ont pas reçu l'approbation de la FDA pour la prévention, le traitement ou la guérison de toute condition médicale, affection ou maladie. Nous devons souligner que toute forme d'introduction corporelle de ces produits chez les humains ou les animaux est strictement interdite par la loi. Il est essentiel de respecter ces directives pour assurer la conformité aux normes légales et éthiques en matière de recherche et d'expérimentation.