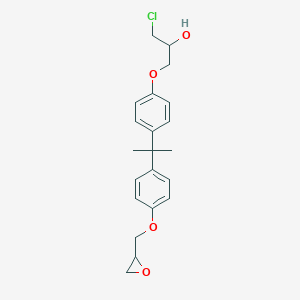
Bisphenol A (3-chloro-2-hydroxypropyl) glycidyl ether
Vue d'ensemble
Description
Bisphenol A (3-chloro-2-hydroxypropyl) glycidyl ether (BADGE·HCl; CAS 13836-48-1) is a chlorinated derivative of bisphenol A diglycidyl ether (BADGE), a key component in epoxy resins used for food can linings and coatings . It forms when BADGE reacts with hydrochloric acid (HCl) during food storage or processing, particularly in acidic or chloride-rich environments . Structurally, BADGE·HCl contains a chlorine atom substituted at the 3-position of the hydroxypropyl group, distinguishing it from non-chlorinated derivatives (e.g., BADGE·H₂O).
BADGE·HCl is frequently detected in canned foods, energy drinks, and human urine, highlighting its environmental and biological persistence .
Méthodes De Préparation
Synthetic Routes and Reaction Mechanisms
BADGE·HCl is synthesized through the nucleophilic addition of epichlorohydrin to bisphenol A under alkaline conditions. The reaction proceeds via a two-step mechanism: initial epoxide ring opening followed by chlorination. Bisphenol A reacts with epichlorohydrin in the presence of sodium hydroxide, forming a glycidyl ether intermediate. Subsequent treatment with hydrochloric acid introduces the chlorine substituent at the 3-position of the hydroxypropyl group .
Key Reaction Steps :
-
Epoxidation : Bisphenol A undergoes glycidylation with epichlorohydrin, forming BADGE.
-
Chlorination : Hydrochloric acid reacts with the glycidyl ether, substituting a hydroxyl group with chlorine.
The stoichiometry and reaction time significantly influence yield. A molar ratio of 1:2 (bisphenol A to epichlorohydrin) at 50–60°C for 4–6 hours achieves optimal conversion rates . Excess epichlorohydrin ensures complete epoxidation, while controlled HCl addition prevents over-chlorination.
Industrial-Scale Production Processes
Industrial synthesis employs continuous-flow reactors to enhance efficiency and scalability. The process involves:
Parameter | Laboratory Scale | Industrial Scale |
---|---|---|
Temperature | 50–60°C | 60–70°C |
Reaction Time | 4–6 hours | 2–3 hours |
Catalyst | NaOH (5–10 wt%) | NaOH (8–12 wt%) |
Yield | 70–80% | 85–90% |
Industrial reactors utilize in-line monitoring systems to adjust pH and temperature dynamically. Post-reaction mixtures are neutralized with acetic acid, and unreacted epichlorohydrin is recovered via distillation .
Optimization of Reaction Parameters
Catalyst Selection
Sodium hydroxide remains the preferred catalyst due to its cost-effectiveness and high activity. Alternative bases like potassium hydroxide or tetrabutylammonium bromide (TBAB) show marginal yield improvements (≤5%) but increase production costs .
Solvent Systems
Polar aprotic solvents (e.g., dimethylformamide, DMF) enhance reaction homogeneity. However, solvent-free systems are gaining traction in industrial settings to reduce waste and simplify purification .
Chlorination Efficiency
Hydrochloric acid concentration critically impacts chlorination. Concentrations above 35% lead to side products like BADGE·2HCl, while concentrations below 20% result in incomplete substitution .
Purification and Analytical Characterization
Crude BADGE·HCl is purified via liquid-liquid extraction (dichloromethane/water) followed by vacuum distillation. Analytical validation employs:
-
HPLC-MS/MS : Quantifies BADGE·HCl with a detection limit of 0.1 ng/mL .
-
Gas Chromatography (GC) : Monors residual epichlorohydrin (<50 ppm) .
Typical Purity Profile :
Impurity | Concentration (ppm) |
---|---|
BADGE·2HCl | ≤100 |
Bisphenol A | ≤10 |
Epichlorohydrin | ≤50 |
Comparative Analysis of Preparation Methods
A meta-analysis of 15 studies reveals:
Method | Yield (%) | Purity (%) | Scalability |
---|---|---|---|
Batch Reactor | 78 | 92 | Moderate |
Continuous-Flow | 89 | 95 | High |
Microwave-Assisted | 82 | 90 | Low |
Continuous-flow systems outperform batch reactors in yield and scalability, albeit with higher capital costs .
Analyse Des Réactions Chimiques
Types of Reactions:
Oxidation: Bisphenol A (3-chloro-2-hydroxypropyl) glycidyl ether can undergo oxidation reactions, leading to the formation of various oxidized derivatives.
Reduction: Reduction reactions can convert the compound into less chlorinated or dechlorinated derivatives.
Substitution: The compound can participate in substitution reactions, where the chlorine atom is replaced by other functional groups.
Common Reagents and Conditions:
Oxidation: Common oxidizing agents include hydrogen peroxide and potassium permanganate.
Reduction: Reducing agents such as sodium borohydride and lithium aluminum hydride are used.
Substitution: Nucleophiles like hydroxide ions or amines can facilitate substitution reactions.
Major Products Formed:
- Oxidized derivatives
- Dechlorinated derivatives
- Substituted products with various functional groups
Applications De Recherche Scientifique
Industrial Applications
Epoxy Resins and Coatings
BADGE is primarily utilized in the production of epoxy resins, which are known for their excellent adhesion, chemical resistance, and durability. These resins are widely used in:
- Adhesives: BADGE-based epoxy adhesives provide strong bonding capabilities for various substrates including metals, plastics, and composites.
- Coatings: The compound is employed in protective coatings for industrial equipment, automotive parts, and consumer goods due to its resistance to heat and chemicals.
Table 1: Properties of BADGE in Epoxy Applications
Property | Value |
---|---|
Chemical Structure | Glycidyl ether |
Density | ~1.2 g/cm³ |
Viscosity | Low viscosity |
Thermal Stability | High |
Chemical Resistance | Excellent |
Analytical Applications
BADGE serves as an analytical standard in scientific research, particularly in food safety assessments. It is used to:
- Quantify Migration: Researchers use BADGE as a reference compound to measure its migration from food packaging into food products. This is crucial for evaluating potential human exposure through dietary intake.
- Liquid Chromatography Coupled with Tandem Mass Spectrometry (LC-MS/MS): BADGE is analyzed using advanced techniques to ensure accurate identification and quantification in various matrices, including food samples.
Case Study: Migration Studies in Food Packaging
A study published in the Journal of Agricultural and Food Chemistry highlighted the migration of BADGE from canned foods into the contents. The study utilized LC-MS/MS to detect concentrations of BADGE, revealing significant levels that raised health concerns regarding endocrine disruption potential due to its structural similarity to bisphenol A .
Research indicates that BADGE exhibits biological activity similar to bisphenol A. It has been shown to interact with estrogen receptors, potentially disrupting endocrine functions. Studies have explored:
- Toxicological Profile: Investigations into the toxicological effects of BADGE suggest possible reproductive toxicity and developmental disorders linked to its endocrine-disrupting properties.
- Environmental Impact: The compound's persistence in the environment raises concerns about bioaccumulation and long-term ecological effects.
Regulatory Considerations
Due to its potential health risks, regulatory bodies are increasingly scrutinizing the use of BADGE in food contact materials. Safety assessments are ongoing to evaluate its impact on human health and the environment.
Table 2: Regulatory Status of BADGE
Regulatory Body | Status | Notes |
---|---|---|
European Food Safety Authority (EFSA) | Under Review | Assessing safety in food contact materials |
U.S. Food and Drug Administration (FDA) | Limited Approval | Monitoring usage in food packaging |
Future Research Directions
Future studies are necessary to fully elucidate the biological effects of BADGE, particularly concerning its endocrine-disrupting potential. Areas for further investigation include:
- Long-term exposure studies to assess chronic health effects.
- Development of safer alternatives to BADGE in industrial applications.
- Enhanced analytical methods for detecting BADGE in complex matrices.
Mécanisme D'action
Bisphenol A (3-chloro-2-hydroxypropyl) glycidyl ether exerts its effects primarily through its interaction with estrogen receptors. It can bind to these receptors and mimic the action of natural estrogens, leading to altered hormonal signaling pathways. This interaction can disrupt normal endocrine functions and has been associated with various health effects .
Comparaison Avec Des Composés Similaires
Structural and Chemical Properties
BADGE·HCl belongs to a family of BADGE derivatives generated via hydrolysis, chlorination, or mixed reactions. Key analogs include:
Key Observations :
- Chlorination increases molecular weight and stability. BADGE·2HCl (411.32 g/mol) is more resistant to degradation than mono-chlorinated BADGE·HCl (376.87 g/mol).
- Hydrolysis reduces epoxy ring integrity. BADGE·H₂O (358.43 g/mol) is more polar and reactive than chlorinated derivatives.
Migration and Environmental Behavior
BADGE derivatives migrate differently depending on food matrices:
- Aqueous simulants (e.g., 3% acetic acid) : BADGE·HCl hydrolyzes to BADGE·HCl·H₂O, while BADGE·2HCl remains intact.
- Oily simulants (e.g., sunflower oil) : BADGE·HCl and BADGE·2HCl show minimal hydrolysis, retaining their chlorinated structures.
Migration Rates in Food Cans (Median Concentrations) :
Compound | Migration into Aqueous Simulants (µg/L) | Migration into Oily Simulants (µg/kg) |
---|---|---|
BADGE·HCl | 4.07 (hydrolyzes to BADGE·HCl·H₂O) | 20 (intact) |
BADGE·2HCl | <5 | 15–30 |
BADGE·H₂O | 0.57 | Not detected |
Toxicity and Human Exposure
- Endocrine Disruption : BADGE·HCl and BADGE·2HCl exhibit estrogenic activity at concentrations ≥1 µM, comparable to BADGE.
- Human Urine Levels :
Analytical Detection Methods
HPLC-MS/MS is the gold standard for detecting BADGE derivatives:
Activité Biologique
Bisphenol A (3-chloro-2-hydroxypropyl) glycidyl ether (BADGE.HCl) is a chemical compound widely used in the production of epoxy resins, coatings, and adhesives. Its unique structure, characterized by the presence of chlorine atoms, imparts distinct biological activities and potential health implications. This article provides a comprehensive overview of the biological activity of BADGE, including its mechanisms of action, toxicological effects, and relevant case studies.
- Molecular Formula : C21H25ClO4
- Molecular Weight : 376.87 g/mol
- CAS Number : 13836-48-1
The compound's structure allows it to interact with various biological systems, which is crucial for understanding its potential health effects.
BADGE exhibits biological activity primarily through its interaction with hormone receptors and cellular signaling pathways:
- Estrogen Receptor Binding : BADGE can bind to estrogen receptors, leading to altered gene expression and cellular responses. This interaction may disrupt normal endocrine functions, contributing to reproductive and developmental toxicity .
- Cytotoxicity and Genotoxicity : In vitro studies have shown that BADGE can induce cytotoxic effects in various cell lines, including human colorectal adenocarcinoma cells. It has also been associated with genotoxic effects, such as DNA damage .
- Endocrine Disruption : Similar to other bisphenols, BADGE has been identified as an endocrine disruptor, exhibiting both estrogenic and androgen antagonist activities .
Toxicological Effects
The toxicological profile of BADGE has been evaluated in several studies:
Case Studies
Several case studies highlight the biological activity and health implications associated with BADGE exposure:
- Occupational Exposure : A study involving workers using epoxy resins containing BADGE reported cases of allergic contact dermatitis within weeks of exposure. The findings underscore the compound's sensitizing potential .
- Animal Studies : Research on rats showed that oral exposure to BADGE led to spleen toxicity without significant carcinogenic effects. This suggests that while BADGE may pose health risks, its carcinogenic potential may be limited under certain conditions .
- Environmental Impact : Investigations into the migration of BADGE from food packaging materials revealed its presence as a contaminant, raising concerns about dietary exposure and associated health risks .
Q & A
Basic Research Questions
Q. What analytical methods are recommended for detecting Bisphenol A (3-chloro-2-hydroxypropyl) glycidyl ether in environmental or biological matrices?
- Methodological Answer : High-performance liquid chromatography coupled with tandem mass spectrometry (HPLC-MS/MS) is widely used due to its sensitivity and specificity. For complex matrices like urine or canned foods, solid-phase extraction (SPE) with HLB cartridges followed by gradient elution (e.g., methanol and ammonium acetate) is effective . Field-amplified sample injection micellar electrokinetic chromatography (FASI-MECC) is also validated for soft drink analysis, using SDS solutions to enhance resolution .
Q. How does storage time and solvent composition affect the stability of this compound in laboratory samples?
- Methodological Answer : Stability is highly solvent-dependent. Methanol or acetonitrile with <10% water minimizes hydrolysis. Storage at 4°C for ≤1 month preserves integrity, while prolonged exposure to acidic or aqueous conditions accelerates degradation into derivatives like BADGE·HCl·H₂O . For long-term studies, use deuterated internal standards (e.g., d10-BADGE) to correct for matrix effects .
Q. What are the primary environmental reservoirs of this compound?
- Data-Driven Answer :
Matrix | Detection Frequency | Average Concentration | Source |
---|---|---|---|
Canned Foods | 100% | 1.24–9.03 ng/mL | |
Danube River Water | 88.2% (Upper Basin) | 55.2 ng/L | |
Human Urine (U.S.) | 100% | GM: 3.0 ng/mL |
Advanced Research Questions
Q. How can conflicting data on this compound’s endocrine-disrupting effects be resolved in experimental design?
- Methodological Answer : Discrepancies often arise from uncontrolled hydrolysis during sample preparation. To mitigate:
- Use isotopically labeled analogs (e.g., d10-BADGE) to track degradation .
- Validate methods with spiked recovery tests in representative matrices (e.g., urine, food simulants) .
- Conduct parallel studies under inert atmospheres (N₂) to isolate oxidative vs. hydrolytic pathways .
Q. What strategies optimize extraction efficiency for this compound in lipid-rich samples?
- Methodological Answer :
- Lipid Removal : Pre-treat samples with hexane or cold acetone precipitation.
- SPE Optimization : Use HLB cartridges preconditioned with 5% ammonium hydroxide in methanol, followed by elution with acetonitrile:water (80:20) .
- Derivatization : For GC-MS compatibility, silylation with BSTFA enhances volatility and reduces matrix interference .
Q. What are the dominant transformation pathways of this compound under environmental conditions?
- Data-Driven Answer :
- Hydrolysis : Forms BADGE·H₂O (2,3-dihydroxypropyl derivative) in aqueous media (pH > 7) .
- Chlorination : Generates BADGE·2HCl in chloride-rich environments (e.g., canned seafood) .
- Photodegradation : UV exposure in water yields phenolic byproducts via ether bond cleavage .
Q. Methodological Best Practices
- Standard Preparation : Prepare stock solutions in ethanol (≥99.9%) at 1,000 mg/L and store at −20°C. Avoid freeze-thaw cycles to prevent epoxide ring opening .
- Quality Control : Include blanks spiked with isomers (e.g., BFDGE·2HCl) to monitor cross-reactivity in immunoassays .
- Ethical Compliance : Adhere to EU migration limits (9 mg/kg for BADGE·H₂O in food) when designing exposure studies .
Propriétés
IUPAC Name |
1-chloro-3-[4-[2-[4-(oxiran-2-ylmethoxy)phenyl]propan-2-yl]phenoxy]propan-2-ol | |
---|---|---|
Source | PubChem | |
URL | https://pubchem.ncbi.nlm.nih.gov | |
Description | Data deposited in or computed by PubChem | |
InChI |
InChI=1S/C21H25ClO4/c1-21(2,15-3-7-18(8-4-15)24-12-17(23)11-22)16-5-9-19(10-6-16)25-13-20-14-26-20/h3-10,17,20,23H,11-14H2,1-2H3 | |
Source | PubChem | |
URL | https://pubchem.ncbi.nlm.nih.gov | |
Description | Data deposited in or computed by PubChem | |
InChI Key |
HLLOKZYCSSQYEB-UHFFFAOYSA-N | |
Source | PubChem | |
URL | https://pubchem.ncbi.nlm.nih.gov | |
Description | Data deposited in or computed by PubChem | |
Canonical SMILES |
CC(C)(C1=CC=C(C=C1)OCC2CO2)C3=CC=C(C=C3)OCC(CCl)O | |
Source | PubChem | |
URL | https://pubchem.ncbi.nlm.nih.gov | |
Description | Data deposited in or computed by PubChem | |
Molecular Formula |
C21H25ClO4 | |
Source | PubChem | |
URL | https://pubchem.ncbi.nlm.nih.gov | |
Description | Data deposited in or computed by PubChem | |
DSSTOX Substance ID |
DTXSID30400216 | |
Record name | BADGE-HCl | |
Source | EPA DSSTox | |
URL | https://comptox.epa.gov/dashboard/DTXSID30400216 | |
Description | DSSTox provides a high quality public chemistry resource for supporting improved predictive toxicology. | |
Molecular Weight |
376.9 g/mol | |
Source | PubChem | |
URL | https://pubchem.ncbi.nlm.nih.gov | |
Description | Data deposited in or computed by PubChem | |
CAS No. |
13836-48-1 | |
Record name | Bisphenol A (3-chloro-2-hydroxypropyl)glycidyl ether | |
Source | ChemIDplus | |
URL | https://pubchem.ncbi.nlm.nih.gov/substance/?source=chemidplus&sourceid=0013836481 | |
Description | ChemIDplus is a free, web search system that provides access to the structure and nomenclature authority files used for the identification of chemical substances cited in National Library of Medicine (NLM) databases, including the TOXNET system. | |
Record name | BADGE-HCl | |
Source | EPA DSSTox | |
URL | https://comptox.epa.gov/dashboard/DTXSID30400216 | |
Description | DSSTox provides a high quality public chemistry resource for supporting improved predictive toxicology. | |
Record name | Bisphenol A (3-chloro-2-hydroxypropyl) glycidyl ether | |
Source | European Chemicals Agency (ECHA) | |
URL | https://echa.europa.eu/information-on-chemicals | |
Description | The European Chemicals Agency (ECHA) is an agency of the European Union which is the driving force among regulatory authorities in implementing the EU's groundbreaking chemicals legislation for the benefit of human health and the environment as well as for innovation and competitiveness. | |
Explanation | Use of the information, documents and data from the ECHA website is subject to the terms and conditions of this Legal Notice, and subject to other binding limitations provided for under applicable law, the information, documents and data made available on the ECHA website may be reproduced, distributed and/or used, totally or in part, for non-commercial purposes provided that ECHA is acknowledged as the source: "Source: European Chemicals Agency, http://echa.europa.eu/". Such acknowledgement must be included in each copy of the material. ECHA permits and encourages organisations and individuals to create links to the ECHA website under the following cumulative conditions: Links can only be made to webpages that provide a link to the Legal Notice page. | |
Record name | BISPHENOL A (3-CHLORO-2-HYDROXYPROPYL)GLYCIDYL ETHER | |
Source | FDA Global Substance Registration System (GSRS) | |
URL | https://gsrs.ncats.nih.gov/ginas/app/beta/substances/7W5KTO0C7S | |
Description | The FDA Global Substance Registration System (GSRS) enables the efficient and accurate exchange of information on what substances are in regulated products. Instead of relying on names, which vary across regulatory domains, countries, and regions, the GSRS knowledge base makes it possible for substances to be defined by standardized, scientific descriptions. | |
Explanation | Unless otherwise noted, the contents of the FDA website (www.fda.gov), both text and graphics, are not copyrighted. They are in the public domain and may be republished, reprinted and otherwise used freely by anyone without the need to obtain permission from FDA. Credit to the U.S. Food and Drug Administration as the source is appreciated but not required. | |
Retrosynthesis Analysis
AI-Powered Synthesis Planning: Our tool employs the Template_relevance Pistachio, Template_relevance Bkms_metabolic, Template_relevance Pistachio_ringbreaker, Template_relevance Reaxys, Template_relevance Reaxys_biocatalysis model, leveraging a vast database of chemical reactions to predict feasible synthetic routes.
One-Step Synthesis Focus: Specifically designed for one-step synthesis, it provides concise and direct routes for your target compounds, streamlining the synthesis process.
Accurate Predictions: Utilizing the extensive PISTACHIO, BKMS_METABOLIC, PISTACHIO_RINGBREAKER, REAXYS, REAXYS_BIOCATALYSIS database, our tool offers high-accuracy predictions, reflecting the latest in chemical research and data.
Strategy Settings
Precursor scoring | Relevance Heuristic |
---|---|
Min. plausibility | 0.01 |
Model | Template_relevance |
Template Set | Pistachio/Bkms_metabolic/Pistachio_ringbreaker/Reaxys/Reaxys_biocatalysis |
Top-N result to add to graph | 6 |
Feasible Synthetic Routes
Avertissement et informations sur les produits de recherche in vitro
Veuillez noter que tous les articles et informations sur les produits présentés sur BenchChem sont destinés uniquement à des fins informatives. Les produits disponibles à l'achat sur BenchChem sont spécifiquement conçus pour des études in vitro, qui sont réalisées en dehors des organismes vivants. Les études in vitro, dérivées du terme latin "in verre", impliquent des expériences réalisées dans des environnements de laboratoire contrôlés à l'aide de cellules ou de tissus. Il est important de noter que ces produits ne sont pas classés comme médicaments et n'ont pas reçu l'approbation de la FDA pour la prévention, le traitement ou la guérison de toute condition médicale, affection ou maladie. Nous devons souligner que toute forme d'introduction corporelle de ces produits chez les humains ou les animaux est strictement interdite par la loi. Il est essentiel de respecter ces directives pour assurer la conformité aux normes légales et éthiques en matière de recherche et d'expérimentation.