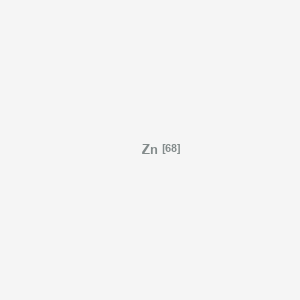
68Zn
Vue d'ensemble
Description
68Zn, is a stable isotope of zinc with an atomic number of 30 and a mass number of 68. It contains 30 protons and 38 neutrons in its nucleus. This isotope is one of the five stable isotopes of zinc, which also include zinc-64, zinc-66, zinc-67, and zinc-70. Zinc-68 has a natural abundance of approximately 18.45% . It is commonly used in various scientific research applications due to its stability and unique properties.
Méthodes De Préparation
Synthetic Routes and Reaction Conditions: Zinc-68 can be produced through the irradiation of zinc-67 in a nuclear reactor. The process involves neutron capture, where zinc-67 absorbs a neutron and transforms into zinc-68. This method requires precise control of neutron flux and irradiation time to ensure the desired isotope is produced efficiently .
Industrial Production Methods: Industrial production of zinc-68 typically involves the use of enriched zinc-67 targets. These targets are placed in a nuclear reactor and subjected to neutron irradiation. The resulting zinc-68 is then separated and purified using chemical processes such as ion exchange chromatography and solvent extraction .
Analyse Des Réactions Chimiques
Types of Reactions: Zinc-68, like other zinc isotopes, can undergo various chemical reactions, including:
Oxidation: Zinc-68 can react with oxygen to form zinc oxide (ZnO).
Reduction: Zinc-68 can be reduced from its ionic form (Zn^2+) to its metallic form (Zn) using reducing agents such as hydrogen gas.
Substitution: Zinc-68 can participate in substitution reactions, where it replaces another metal in a compound.
Common Reagents and Conditions:
Oxidation: Typically involves heating zinc-68 in the presence of oxygen or air.
Reduction: Commonly performed using hydrogen gas or other reducing agents at elevated temperatures.
Substitution: Often carried out in aqueous solutions with appropriate ligands and catalysts.
Major Products Formed:
Oxidation: Zinc oxide (ZnO)
Reduction: Metallic zinc (Zn)
Substitution: Various zinc-containing compounds depending on the reactants used.
Applications De Recherche Scientifique
Zinc-68 has a wide range of applications in scientific research, including:
Chemistry: Used as a tracer in studies of zinc’s chemical behavior and reaction mechanisms.
Biology: Employed in studies of zinc’s role in biological systems, including enzyme function and cellular processes.
Medicine: Utilized in medical imaging and diagnostic techniques, such as positron emission tomography (PET) scans.
Industry: Applied in the development of new materials and in the study of zinc’s properties in various industrial processes
Mécanisme D'action
The mechanism by which zinc-68 exerts its effects is primarily related to its role as a structural and catalytic component in various biological and chemical systems. In biological systems, zinc-68 can bind to proteins and enzymes, influencing their structure and function. It can also participate in redox reactions, acting as an electron donor or acceptor. The pathways involved in these processes include zinc finger proteins, metalloproteins, and various enzymatic reactions .
Comparaison Avec Des Composés Similaires
Zinc-64: Another stable isotope of zinc with a higher natural abundance (49.17%).
Zinc-66: A stable isotope with a natural abundance of 27.73%.
Zinc-67: A stable isotope with a lower natural abundance (4.04%).
Zinc-70: The least abundant stable isotope of zinc (0.61%).
Comparison: Zinc-68 is unique due to its specific neutron-to-proton ratio, which gives it distinct nuclear properties compared to other zinc isotopes. Its stability and moderate natural abundance make it particularly useful in scientific research, especially in studies requiring precise isotopic measurements. Unlike zinc-64, which is more abundant, zinc-68 offers a balance between availability and specificity for certain applications .
Propriétés
IUPAC Name |
zinc-68 | |
---|---|---|
Source | PubChem | |
URL | https://pubchem.ncbi.nlm.nih.gov | |
Description | Data deposited in or computed by PubChem | |
InChI |
InChI=1S/Zn/i1+3 | |
Source | PubChem | |
URL | https://pubchem.ncbi.nlm.nih.gov | |
Description | Data deposited in or computed by PubChem | |
InChI Key |
HCHKCACWOHOZIP-AKLPVKDBSA-N | |
Source | PubChem | |
URL | https://pubchem.ncbi.nlm.nih.gov | |
Description | Data deposited in or computed by PubChem | |
Canonical SMILES |
[Zn] | |
Source | PubChem | |
URL | https://pubchem.ncbi.nlm.nih.gov | |
Description | Data deposited in or computed by PubChem | |
Isomeric SMILES |
[68Zn] | |
Source | PubChem | |
URL | https://pubchem.ncbi.nlm.nih.gov | |
Description | Data deposited in or computed by PubChem | |
Molecular Formula |
Zn | |
Source | PubChem | |
URL | https://pubchem.ncbi.nlm.nih.gov | |
Description | Data deposited in or computed by PubChem | |
DSSTOX Substance ID |
DTXSID60932090 | |
Record name | (~68~Zn)Zinc | |
Source | EPA DSSTox | |
URL | https://comptox.epa.gov/dashboard/DTXSID60932090 | |
Description | DSSTox provides a high quality public chemistry resource for supporting improved predictive toxicology. | |
Molecular Weight |
67.924844 g/mol | |
Source | PubChem | |
URL | https://pubchem.ncbi.nlm.nih.gov | |
Description | Data deposited in or computed by PubChem | |
CAS No. |
14378-35-9 | |
Record name | Zinc, isotope of mass 68 | |
Source | ChemIDplus | |
URL | https://pubchem.ncbi.nlm.nih.gov/substance/?source=chemidplus&sourceid=0014378359 | |
Description | ChemIDplus is a free, web search system that provides access to the structure and nomenclature authority files used for the identification of chemical substances cited in National Library of Medicine (NLM) databases, including the TOXNET system. | |
Record name | (~68~Zn)Zinc | |
Source | EPA DSSTox | |
URL | https://comptox.epa.gov/dashboard/DTXSID60932090 | |
Description | DSSTox provides a high quality public chemistry resource for supporting improved predictive toxicology. | |
Synthesis routes and methods I
Procedure details
Synthesis routes and methods II
Procedure details
Synthesis routes and methods III
Procedure details
Synthesis routes and methods IV
Procedure details
Avertissement et informations sur les produits de recherche in vitro
Veuillez noter que tous les articles et informations sur les produits présentés sur BenchChem sont destinés uniquement à des fins informatives. Les produits disponibles à l'achat sur BenchChem sont spécifiquement conçus pour des études in vitro, qui sont réalisées en dehors des organismes vivants. Les études in vitro, dérivées du terme latin "in verre", impliquent des expériences réalisées dans des environnements de laboratoire contrôlés à l'aide de cellules ou de tissus. Il est important de noter que ces produits ne sont pas classés comme médicaments et n'ont pas reçu l'approbation de la FDA pour la prévention, le traitement ou la guérison de toute condition médicale, affection ou maladie. Nous devons souligner que toute forme d'introduction corporelle de ces produits chez les humains ou les animaux est strictement interdite par la loi. Il est essentiel de respecter ces directives pour assurer la conformité aux normes légales et éthiques en matière de recherche et d'expérimentation.