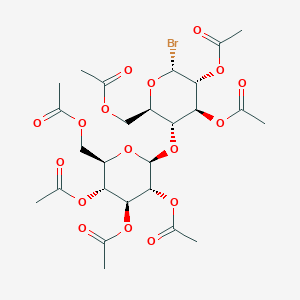
alpha-D-Cellobiosyl bromide heptaacetate
Vue d'ensemble
Description
Synthesis Analysis
The commercial synthesis process for alpha-D-cellobiosyl bromide heptaacetate involves the reaction of alpha-D-cellobiose octaacetate (CBO) with hydrogen bromide (HBr) in glacial acetic acid or a combination of glacial acetic acid and methylene chloride, yielding the target compound in high yield and excellent quality. The process variables optimized for large-scale synthesis include reaction solvent, time, temperature, HBr stoichiometry, isolation methods, and product purification options, enabling successful preparation in a commercial manufacturing setting (Goggin et al., 1994).
Molecular Structure Analysis
The molecular structure of alpha-D-cellobiosyl bromide heptaacetate, particularly in complex forms like with NaI and water, reveals intricate details about its conformation and interaction potential. In such a complex, direct hydrogen bonds among cellobiose molecules are absent, with intramolecular hydrogen bonding patterns significantly influenced by the presence of Na+ ions. These structural insights are crucial for understanding how the compound interacts in chemical reactions and its role in forming glycosidic bonds (Peralta-Inga et al., 2002).
Chemical Reactions and Properties
Alpha-D-Cellobiosyl bromide heptaacetate participates in various chemical reactions, serving as a key intermediate in the synthesis of glycosides and oligosaccharides. Its reactivity under different conditions, including with lithium chloride to form glycosyl chloride derivatives, showcases its versatility in synthetic chemistry. Such reactions are fundamental in the synthesis of complex carbohydrates and glycoconjugates, underscoring the compound's significant utility in glycoscience (Dick & Wesleder, 1976).
Applications De Recherche Scientifique
Commercial Synthesis : Alpha-D-cellobiosyl bromide heptaacetate has been developed for commercial synthesis with optimized process variables like reaction solvent, time, temperature, and methods for isolation and purification. Its synthesis involves the reaction of alpha-D-cellobiose octaacetate with HBr in glacial acetic acid, yielding high-quality alpha-D-cellobiosyl bromide heptaacetate in high yield. This development has been crucial for large-scale production and subsequent applications in various scientific domains (Goggin et al., 1994).
Synthesis of Glycosyl Chloride Derivatives : The compound has been used to create 1,2-trans-O-acetyl glycosyl chloride derivatives of d-glucose, cellobiose, and lactose. This process involves converting O-acetyl-α-glycosyl bromide derivatives into corresponding β-chlorides, demonstrating the compound's versatility and utility in synthesizing various glycosidic linkages and derivatives (Dick & Wesleder, 1976).
Thermal Glycosylations : Beta-cellobiosyl fluoride heptaacetate, a related compound, has been utilized in thermal reactions with other molecules like tigogenin in organic solvents. This process avoids the use of traditional glycosidation promotors and Lewis acids, indicating the potential for alpha-D-cellobiosyl bromide heptaacetate in similar thermal glycosylation reactions (Goggin, Lambert & Walinsky, 1997).
Hydrolysis and Enzymatic Studies : Studies on the hydrolysis of alpha- and beta-cellobiosyl fluorides by enzymes like cellobiohydrolases have provided insights into the mechanism of glycosidic bond cleavage and enzyme specificity. Such studies could be relevant for understanding the reactivity and potential enzymatic applications of alpha-D-cellobiosyl bromide heptaacetate (Konstantinidis, Marsden & Sinnott, 1993).
Synthesis of Glycopeptide Analogues : The compound has potential applications in the synthesis of glycopeptide analogues, where glycosidic bromides are used with amino acids to create glycosylated peptides. This can be particularly useful in studying the role of glycosylation in peptide and protein function (Mitchell et al., 2001).
Mécanisme D'action
Target of Action
Alpha-D-Cellobiosyl bromide heptaacetate, also known as Cel Br heptaacetate, is a biochemical reagent . It can be used as a biological material or organic compound for life science related research . .
Mode of Action
As a biochemical reagent, it likely interacts with its targets to induce certain biochemical reactions or changes
Biochemical Pathways
As a biochemical reagent, it may be involved in various biochemical reactions or pathways depending on the specific context of its use .
Pharmacokinetics
As a biochemical reagent, its bioavailability would likely depend on the specific conditions of its use, including the method of administration, the presence of other compounds, and the physiological state of the organism .
Result of Action
As a biochemical reagent, it likely induces certain changes at the molecular and cellular level, but the specifics would depend on the context of its use .
Propriétés
IUPAC Name |
[(2R,3R,4S,5R,6S)-3,4,5-triacetyloxy-6-[(2R,3R,4S,5R,6R)-4,5-diacetyloxy-2-(acetyloxymethyl)-6-bromooxan-3-yl]oxyoxan-2-yl]methyl acetate | |
---|---|---|
Source | PubChem | |
URL | https://pubchem.ncbi.nlm.nih.gov | |
Description | Data deposited in or computed by PubChem | |
InChI |
InChI=1S/C26H35BrO17/c1-10(28)35-8-17-20(21(38-13(4)31)23(25(27)42-17)40-15(6)33)44-26-24(41-16(7)34)22(39-14(5)32)19(37-12(3)30)18(43-26)9-36-11(2)29/h17-26H,8-9H2,1-7H3/t17-,18-,19-,20-,21+,22+,23-,24-,25+,26+/m1/s1 | |
Source | PubChem | |
URL | https://pubchem.ncbi.nlm.nih.gov | |
Description | Data deposited in or computed by PubChem | |
InChI Key |
NLFHLQWXGDPOME-VRECAULFSA-N | |
Source | PubChem | |
URL | https://pubchem.ncbi.nlm.nih.gov | |
Description | Data deposited in or computed by PubChem | |
Canonical SMILES |
CC(=O)OCC1C(C(C(C(O1)OC2C(OC(C(C2OC(=O)C)OC(=O)C)Br)COC(=O)C)OC(=O)C)OC(=O)C)OC(=O)C | |
Source | PubChem | |
URL | https://pubchem.ncbi.nlm.nih.gov | |
Description | Data deposited in or computed by PubChem | |
Isomeric SMILES |
CC(=O)OC[C@@H]1[C@H]([C@@H]([C@H]([C@@H](O1)O[C@@H]2[C@H](O[C@@H]([C@@H]([C@H]2OC(=O)C)OC(=O)C)Br)COC(=O)C)OC(=O)C)OC(=O)C)OC(=O)C | |
Source | PubChem | |
URL | https://pubchem.ncbi.nlm.nih.gov | |
Description | Data deposited in or computed by PubChem | |
Molecular Formula |
C26H35BrO17 | |
Source | PubChem | |
URL | https://pubchem.ncbi.nlm.nih.gov | |
Description | Data deposited in or computed by PubChem | |
DSSTOX Substance ID |
DTXSID70931397 | |
Record name | 2,3,6-Tri-O-acetyl-4-O-(2,3,4,6-tetra-O-acetylhexopyranosyl)hexopyranosyl bromide | |
Source | EPA DSSTox | |
URL | https://comptox.epa.gov/dashboard/DTXSID70931397 | |
Description | DSSTox provides a high quality public chemistry resource for supporting improved predictive toxicology. | |
Molecular Weight |
699.4 g/mol | |
Source | PubChem | |
URL | https://pubchem.ncbi.nlm.nih.gov | |
Description | Data deposited in or computed by PubChem | |
Product Name |
alpha-D-Cellobiosyl bromide heptaacetate | |
CAS RN |
14227-66-8 | |
Record name | Cellobiosyl bromide heptaacetate | |
Source | ChemIDplus | |
URL | https://pubchem.ncbi.nlm.nih.gov/substance/?source=chemidplus&sourceid=0014227668 | |
Description | ChemIDplus is a free, web search system that provides access to the structure and nomenclature authority files used for the identification of chemical substances cited in National Library of Medicine (NLM) databases, including the TOXNET system. | |
Record name | 2,3,6-Tri-O-acetyl-4-O-(2,3,4,6-tetra-O-acetylhexopyranosyl)hexopyranosyl bromide | |
Source | EPA DSSTox | |
URL | https://comptox.epa.gov/dashboard/DTXSID70931397 | |
Description | DSSTox provides a high quality public chemistry resource for supporting improved predictive toxicology. | |
Retrosynthesis Analysis
AI-Powered Synthesis Planning: Our tool employs the Template_relevance Pistachio, Template_relevance Bkms_metabolic, Template_relevance Pistachio_ringbreaker, Template_relevance Reaxys, Template_relevance Reaxys_biocatalysis model, leveraging a vast database of chemical reactions to predict feasible synthetic routes.
One-Step Synthesis Focus: Specifically designed for one-step synthesis, it provides concise and direct routes for your target compounds, streamlining the synthesis process.
Accurate Predictions: Utilizing the extensive PISTACHIO, BKMS_METABOLIC, PISTACHIO_RINGBREAKER, REAXYS, REAXYS_BIOCATALYSIS database, our tool offers high-accuracy predictions, reflecting the latest in chemical research and data.
Strategy Settings
Precursor scoring | Relevance Heuristic |
---|---|
Min. plausibility | 0.01 |
Model | Template_relevance |
Template Set | Pistachio/Bkms_metabolic/Pistachio_ringbreaker/Reaxys/Reaxys_biocatalysis |
Top-N result to add to graph | 6 |
Feasible Synthetic Routes
Q & A
Q1: What is the significance of developing a commercial synthesis method for α-D-Cellobiosyl bromide heptaacetate?
A1: α-D-Cellobiosyl bromide heptaacetate is a valuable building block in carbohydrate chemistry. It acts as an activated donor of the cellobiose disaccharide unit. Having a commercially viable synthesis method [, ] makes this compound more accessible for researchers and industries working on various applications, including the synthesis of complex carbohydrates, glycoconjugates, and other materials.
Q2: What are the key process parameters that were optimized during the development of the commercial synthesis method?
A2: The research highlights several key process parameters that were optimized for large-scale production of α-D-Cellobiosyl bromide heptaacetate [, ]. These include:
Q3: What is the significance of successfully producing α-D-Cellobiosyl bromide heptaacetate in a commercial manufacturing plant?
A3: Successfully scaling up the synthesis from a laboratory setting to a commercial manufacturing plant [, ] demonstrates the feasibility of producing this important compound in larger quantities. This achievement paves the way for wider availability and potential cost reductions, benefiting researchers and industries utilizing α-D-Cellobiosyl bromide heptaacetate in their work.
Avertissement et informations sur les produits de recherche in vitro
Veuillez noter que tous les articles et informations sur les produits présentés sur BenchChem sont destinés uniquement à des fins informatives. Les produits disponibles à l'achat sur BenchChem sont spécifiquement conçus pour des études in vitro, qui sont réalisées en dehors des organismes vivants. Les études in vitro, dérivées du terme latin "in verre", impliquent des expériences réalisées dans des environnements de laboratoire contrôlés à l'aide de cellules ou de tissus. Il est important de noter que ces produits ne sont pas classés comme médicaments et n'ont pas reçu l'approbation de la FDA pour la prévention, le traitement ou la guérison de toute condition médicale, affection ou maladie. Nous devons souligner que toute forme d'introduction corporelle de ces produits chez les humains ou les animaux est strictement interdite par la loi. Il est essentiel de respecter ces directives pour assurer la conformité aux normes légales et éthiques en matière de recherche et d'expérimentation.